
- Opto-Electronic Advances
- Vol. 4, Issue 2, 200031-1 (2021)
Abstract
Introduction
Ultrathin flat diffractive optical devices with subwavelength thickness, such as metalenses, have emerged as promising alternatives to conventional diffractive devices, which offer new opportunities for myriads of miniaturization and interfacial applications, such as visual reality/augmented reality helmet-mounted display systems, miniaturized cameras
Nowadays, due to the increasing demands on integratable optical components, the thickness of flat lenses needs to be reduced significantly, which inevitably challenges the design and fabrication techniques. Therefore, it is desired to achieve ultrathin flat metalenses design, which modulates the phase and amplitude of the incident beam simultaneously for high focusing performance with subwavelength thickness. Although, focusing and holograms have been achieved using phase only modulation in previous works, considering practical applications and sampling theory, controlling both phase and amplitude of the electric field allows more flexibilities in the design, thus it is easier to achieve more accurate light intensity distributions for tighter focal spots and holograms of higher quality
Alternatively, flat lenses can be designed based on nanostructure engineering such as plasmonic nanostructures
Result and discussion
Recently, high index dielectric materials, like GaN, ZnO and TiO2, have been widely used in the field of metasurface and metalens, due to their sufficient wavefront modulation capability, small intrinsic loss in visible range and compatibility with Complementary Metal-Oxide-Semiconductor (CMOS) technology
Figure 1.
A planewave incidents on a glass substrate with a refractive index n1 and passes through the GO metalens with a thickness of t and a complex refractive index of
The focusing schemes of the GO metalenses are shown in Figs. 1(a) and 1(b), in which a planewave incident light beam is focused by the GO metalenses into a super-resolved optical needle and an axial multifocal spot array, respectively. We choose these two cases because they require very accurate phase and amplitude modulations both in design and fabrication, in which the results are extremely sensitive to design/fabrication imperfections. Although the axicon for focusing an ultralong focal spot has been demonstrated by metalens before
The schematic demonstration of the GO metalenses is shown in Fig. 1(c), which is composed of concentric RGO rings and GO rings. The RGO rings are formed by direct femtosecond laser reduction on a uniform GO film, in which process the absorption and the refractive index increase while the thickness reduces
The phase-amplitude dependence is illustrated in Fig. 1(d). The blue line and brown line are the phase-amplitude relationships for the GO and RGO at the wavelength of 632.8 nm, respectively. Note that the maximum thickness change (
where, ϕ and Amp are the phase and amplitude of GO/RGO, respectively. C is the parametric equation of thickness
The position and the linewidth of each ring can be flexibly manipulated to optimize the light interference in the focal region of the incident beam. The linewidth of the rings is fixed at 400 nm, which is decided by the resolution of the laser fabrication system. Based on our quantitative phase-amplitude dependency, the optical needle lens and the multifocal lens are designed using an optimization process, in which the positions of the rings are input variables; the desired focal region optical field is the target function (see Methods).
The designed lenses (Supplementary information Table S2 and Table S3) are fabricated by reducing the GO film with direct laser writing (DLW), the optical setup of which is shown in Fig. S4. The optical image of the multifocal lens is shown in Fig. 2(a), which has a radius of around 180 µm composing of 100 rings. By magnifying the structure with a scanning electron microscope (SEM) (Fig. 2(b)), the 400 nm linewidth by laser reduction is verified. In order to visualize the morphology of the lens, a three-dimensional (3D) optical profiler (Bruker ContourGT InMotion) (Fig. 2(c)) and an AFM (Fig. 2(d)) were used. The results indicate that the thickness is reduced, and the Gaussian like profiles resulting from the laser reduction can be clearly identified (indicated by the cross-sectional plot in Fig. 2(d)), which is similar to our design in Fig. S3(a). The maximum
Figure 2.
The focusing performance is characterized by a homemade imaging system (Fig. S5) consisting of a high NA objective (NA=0.8, 100×) mounted on a nanometric piezo stage and a tube lens (f = 200 mm). The intensity distribution in the focal region is captured by a high dynamic range (HDR) charge coupled device (CCD) camera. The lens is illuminated by the collimated planewave from a He-Ne laser at 632.8 nm.
The theoretical result of the optical needle in the x-z plane is shown in Fig. 3(a), in which a needle with a subwavelength full width at half maximum (FWHM) along the lateral direction (Fig. 3(d)) covering the axial range of 16.6λ (10.5 μm) in designed (Fig. 3(c)). The corresponding experimental intensity distributions in the x-z plane and lateral FWHM are shown in Figs. 3(b) and 3(d), which fit well with the simulation results. In Fig. 3(c), both theoretical and experimental normalized intensity along the optical axis are depicted with little discrepancy. Furthermore, normalized intensities at corresponding positions shown in Figs. 3(b) (marked by the white dash lines) are demonstrated in Figs. 3(e)-3(h), and the lateral FWHMs at these positions are 0.81λ, 0.74λ, 0.73λ, 0.70λ, respectively (Fig. 3(d)). The conventional diffraction-limited lateral resolution and axial resolution are
Figure 3.
In addition, the theoretical and experimental results of the axial multifocal array in the x-z plane with four nearly identical focal spots along the optical axis are shown in Figs. 4(a) and 4(b). The lateral and axial resolutions of each spot are ~0.7λ and ~4λ, respectively. The experimentally measured normalized intensity distribution along the z-direction is shown in Fig. 4(c), which agrees well with the theoretical result in the same plot. The Figs. 4(d)-4(g) show that the four focal spot distributions are smooth and nearly identical at the positions marked by dashed lines in Fig. 4(b), with lateral FWHMs of 0.97λ, 0.94λ, 0.87λ, 0.91λ, respectively. It is worth mentioning that the well-separated focal spots with uniform intensity distributions can only be achieved by accurate phase and amplitude modulations, which can be attributed to the accurate control of the complex refractive index and the thickness with the laser reduction technique. Here, with quantitative phase-amplitude dependency guiding our design and laser fabrication, we demonstrate the generation of multifocal arrays with high qualities in the case of using GO metalenses. Although some discrepancy between simulation and experiments can be seen in Fig. 3 and Fig. 4 due to the surface roughness of the as-prepared GO films (the detailed analysis can be found in Supplementary information Section S1.3), the super-resolved optical needle and overall multiple foci distribution are still reasonably well matching the simulation to verify the accuracy of our amplitude and phase dependency and the design of our GO metalenses.
Figure 4.
It is noteworthy that the GO metalenses are thicker than the metasurface designs based on metal structure. However, as metals are general lossy materials in visible range, the efficiency of the metal based design is limited to around 1%
In our previous work we have proved that by using the simultaneous phase and amplitude modulations provided by photo-reduction of GO materials
Conclusion
In conclusion, based on the unique optical properties of graphene-based materials (GO and RGO) under laser illumination, including the complex refractive index and the thickness change, we build up the quantitative phase-amplitude dependency, which provides a well-guided design process for photonic devices based on graphene-based materials with simultaneous amplitude and phase modulations at a single position. By understanding the phase-amplitude dependency, we are able to design photonic devices with a high accuracy. To validate the accuracy of our GO metalens design strategy, we experimentally demonstrated GO metalenses, which generate an ultra-long (~16λ) super-resolved optical needle and an axial multifocal array (4 focal spots) as examples. Although we have demonstrated only two designs in the study, the phase-amplitude dependency can be further applied to design other photonic devices such as optical chain, optical cage, optical bubble and optical tweezers for the applications in optical trapping, optical manipulating, optical delivering and optical staking
Due to the flexibility and mechanical robustness of graphene-based materials as well as the simple, low-cost, and scalable fabrication of GO metalenses, these ultrathin flat optical components enable great potential in applications and possess commercial value in integrated optical device
Methods
Preparation of GO film
The ~200 nm homogeneous GO film was prepared using the vacuum filtration method with high quality GO solution synthesized by the modified Hummer’s method
Lens design and optimization
The designs of the optical needle lens and the multifocal lens are based on the powerful interior-point optimization algorithm
where,
The target function of multifoci is a summation of triangular function along the Z axis defined from 189.90 μm (300λ) to 227.88 μm (360λ).
where, amax is the radius of the largest ring, k is the wavenumber. AGO and φGO are the amplitude and phase modulations for GO in our design, respectively. Meanwhile, AT and φT are the amplitude and phase modulations of RGO zones, which obey our GO phase-amplitude dependency (chromatic transitional route 1 in Fig. 1(b)). The intensity at the focal point is the square of the absolute value of the electric field.
The constrains of the optimization are shown in Eq. (5):
where,
Femtosecond laser fabrication
With a commercial laser 3D nanoprinting setup (Special Edition, Innofocus Nanoprint3D) (Fig. S4), the laser reduction process was performed on our as-prepared GO film according to the radii in supplementary information Tables 1 and 2. The femtosecond laser beam (100 fs pulse, 100 kHz, 800 nm) with a fabrication speed at 30 μm/s was calibrated at 12 μW for reducing the GO film, which is mounted on a 3D nanometric piezo stage (Physik Instrumente). A high NA oil objective (NA=1.4, 100×) is used in fabrication. The lateral resolution of laser fabrication on GO thin film is determined by the size of femtosecond laser focal spot and the laser interaction threshold with the GO material. The FWHM of the laser focal spot is determined by
References
[1] A Arbabi, E Arbabi, SM Kamali, Y Horie, S Han, et al. Miniature optical planar camera based on a wide-angle metasurface doublet corrected for monochromatic aberrations. Nat Commun, 7, 13682(2016).
[2] B Groever, WT Chen, F Capasso. Meta-lens doublet in the visible region. Nano Lett, 17, 4902-4907(2017).
[3] M Farmahini-Farahani, JR Cheng, H Mosallaei. Metasurfaces nanoantennas for light processing. J Opt Soc Am B, 30, 2365-2370(2013).
[4] NF Yu, F Aieta, P Genevet, MA Kats, Z Gaburro, et al. A broadband, background-free quarter-wave plate based on plasmonic metasurfaces. Nano Lett, 12, 6328-6333(2012).
[5] XQ Zhang, Z Tian, WS Yue, JQ Gu, S Zhang, et al. Broadband terahertz wave deflection based on c-shape complex metamaterials with phase discontinuities. Adv Mater, 25, 4567-4572(2013).
[6] P Genevet, NF Yu, F Aieta, J Lin, MA Kats, et al. Ultra-thin plasmonic optical vortex plate based on phase discontinuities. Appl Phys Lett, 100, 013101(2012).
[7] MA Kats, P Genevet, G Aoust, NF Yu, R Blanchard, et al. Giant birefringence in optical antenna arrays with widely tailorable optical anisotropy. Proc Natl Acad Sci USA, 109, 12364-12368(2012).
[8] F Monticone, NM Estakhri, A Alù. Full control of nanoscale optical transmission with a composite metascreen. Phys Rev Lett, 110, 203903(2013).
[9] C Pfeiffer, A Grbic. Metamaterial huygens' surfaces: tailoring wave fronts with reflectionless sheets. Phys Rev Lett, 110, 197401(2013).
[10] NF Yu, P Genevet, MA Kats, F Aieta, JP Tetienne, et al. Light propagation with phase discontinuities: generalized laws of reflection and refraction. Science, 334, 333-337(2011).
[11] K Keskinbora, C Grévent, M Bechtel, M Weigand, E Goering, et al. Ion beam lithography for Fresnel zone plates in X-ray microscopy. Opt Express, 21, 11747-11756(2013).
[12] T Jwad, SA Deng, H Butt, S Dimov. Fabrication of TiO2 thin film-based fresnel zone plates by nanosecond laser direct writing. J Micro Nano-Manuf, 6, 011001(2018).
[13] D Bowman, TL Harte, V Chardonnet, Groot De, SJ Denny, et al. High-fidelity phase and amplitude control of phase-only computer generated holograms using conjugate gradient minimisation. Opt Express, 25, 11692-11700(2017).
[14] GY Lee, G Yoon, SY Lee, H Yun, J Cho, et al. Complete amplitude and phase control of light using broadband holographic metasurfaces. Nanoscale, 10, 4237-4245(2018).
[15] LX Liu, XQ Zhang, M Kenney, XQ Su, NN Xu, et al. Broadband metasurfaces with simultaneous control of phase and amplitude. Adv Mater, 26, 5031-5036(2014).
[16] Q Wang, XQ Zhang, YH Xu, JQ Gu, YF Li, et al. Broadband metasurface holograms: toward complete phase and amplitude engineering. Sci Rep, 6, 32867(2016).
[17] G Yoon, D Lee, KT Nam, J Rho. “Crypto-display” in dual-mode metasurfaces by simultaneous control of phase and spectral responses. ACS Nano, 12, 6421-6428(2018).
[18] C Pfeiffer, C Zhang, V Ray, LJ Guo, A Grbic. High performance bianisotropic metasurfaces: asymmetric transmission of light. Phys Rev Lett, 113, 023902(2014).
[19] ML Tseng, HH Hsiao, CH Chu, MK Chen, G Sun, et al. Metalenses: advances and applications. Adv Opt Mater, 6, 1800554(2018).
[20] J Engelberg, U Levy. The advantages of metalenses over diffractive lenses. Nat Commun, 11, 1991(2020).
[21] SM Wang, PC Wu, VC Su, YC Lai, MK Chen, et al. A broadband achromatic metalens in the visible. Nat Nanotechnol, 13, 227-232(2018).
[22] RH Lin, XH Li. Multifocal metalens based on multilayer pancharatnam-berry phase elements architecture. Opt Lett, 44, 2819-2822(2019).
[23] ML Tseng, YW Huang, MK Hsiao, HW Huang, HM Chen, et al. Fast fabrication of a Ag nanostructure substrate using the femtosecond laser for broad-band and tunable plasmonic enhancement. ACS Nano, 6, 5190-5197(2012).
[24] CH Chu, ML Tseng, Shiue da, SW Chen, HP Chiang, et al. Fabrication of phase-change Ge2Sb2Te5 nano-rings. Opt Express, 19, 12652-12657(2011).
[25] N Lassaline, R Brechbühler, SJW Vonk, K Ridderbeek, M Spieser, et al. Optical fourier surfaces. Nature, 582, 506-510(2020).
[26] KTP Lim, HL Liu, YJ Liu, JKW Yang. Holographic colour prints for enhanced optical security by combined phase and amplitude control. Nat Commun, 10, 25(2019).
[27] WH Yang, SM Xiao, QH Song, YL Liu, YK Wu, et al. All-dielectric metasurface for high-performance structural color. Nat Commun, 11, 1864(2020).
[28] M Semmlinger, ML Tseng, J Yang, M Zhang, C Zhang, et al. Vacuum ultraviolet light-generating metasurface. Nano Lett, 18, 5738-5743(2018).
[29] BH Chen, PC Wu, VC Su, YC Lai, CH Chu, et al. GaN metalens for pixel-level full-color routing at visible light. Nano Lett, 17, 6345-6352(2017).
[30] XW Chen, X Hai, JH Wang. Graphene/graphene oxide and their derivatives in the separation/isolation and preconcentration of protein species: a review. Anal Chim Acta, 922, 1-10(2016).
[31] XR Zheng, BH Jia, H Lin, L Qiu, D Li, et al. Highly efficient and ultra-broadband graphene oxide ultrathin lenses with three-dimensional subwavelength focusing. Nat Commun, 6, 8433(2015).
[32] YY Yang, H Lin, BY Zhang, YN Zhang, XR Zheng, et al. Graphene-based multilayered metamaterials with phototunable architecture for on-chip photonic devices. ACS Photonics, 6, 1033-1040(2019).
[33] TS Yang, H Lin, XR Zheng, KP Loh, BH Jia. Tailoring pores in graphene-based materials: from generation to applications. J Mater Chem A, 5, 16537-16558(2017).
[34] CL Hao, ZQ Nie, HP Ye, H Li, Y Luo, et al. Three-dimensional supercritical resolved light-induced magnetic holography. Sci Adv, 3, e1701398(2017).
[35] H Lin, BCP Sturmberg, KT Lin, YY Yang, XR Zheng, et al. A 90-nm-thick graphene metamaterial for strong and extremely broadband absorption of unpolarized light. Nat Photonics, 13, 270-276(2019).
[36] GY Cao, H Lin, S Fraser, XR Zheng, Rosal Del, et al. Resilient graphene ultrathin flat lens in aerospace, chemical, and biological harsh environments. ACS Appl Mater Interfaces, 11, 20298-20303(2019).
[37] PP Mondal, A Diaspro. Simultaneous multilayer scanning and detection for multiphoton fluorescence microscopy. Sci Rep, 1, 149(2011).
[38] XP Li, YY Cao, M Gu. Superresolution-focal-volume induced 3.0 Tbytes/disk capacity by focusing a radially polarized beam. Opt Lett, 36, 2510-2512(2011).
[39] JM Auñón, CW Qiu, M Nieto-Vesperinas. Tailoring photonic forces on a magnetodielectric nanoparticle with a fluctuating optical source. Phys Rev A, 88, 043817(2013).
[40] J Godin, CH Chen, SH Cho, W Qiao, F Tsai, et al. Microfluidics and photonics for bio-system-on-a-chip: a review of advancements in technology towards a microfluidic flow cytometry chip. J Biophotonics, 1, 355-376(2008).
[41] M Born, E Wolf. Principles of Optics: Electromagnetic Theory of Propagation, Interference and Diffraction of Light(1999).
[42] F Aieta, P Genevet, MA Kats, NF Yu, R Blanchard, et al. Aberration-free ultrathin flat lenses and axicons at telecom wavelengths based on plasmonic metasurfaces. Nano Lett, 12, 4932-4936(2012).
[43] G Mínguez-Vega, J Lancis, J Caraquitena, V Torres-Company, P Andrés. High spatiotemporal resolution in multifocal processing with femtosecond laser pulses. Opt Lett, 31, 2631-2633(2006).
[44] H Lin, BH Jia, M Gu. Dynamic generation of debye diffraction-limited multifocal arrays for direct laser printing nanofabrication. Opt Lett, 36, 406-408(2011).
[45] M Gu, H Lin, XP Li. Parallel multiphoton microscopy with cylindrically polarized multifocal arrays. Opt Lett, 38, 3627-3630(2013).
[46] H Lin, M Gu. Creation of diffraction-limited non-Airy multifocal arrays using a spatially shifted vortex beam. Appl Phys Lett, 102, 084103(2013).
[47] LW Zhu, JJ Yu, DW Zhang, MY Sun, JN Chen. Multifocal spot array generated by fractional Talbot effect phase-only modulation. Opt Express, 22, 9798-9808(2014).
[48] ZQ Nie, H Lin, XF Liu, AP Zhai, YT Tian, et al. Three-dimensional super-resolution longitudinal magnetization spot arrays. Light Sci Appl, 6, e17032(2017).
[49] XF Zang, HZ Ding, Y Intaravanne, L Chen, Y Peng, et al. A multi-foci metalens with polarization-rotated focal points. Laser Photon Rev, 13, 1900182(2019).
[50] MQ Mehmood, H Liu, K Huang, ST Mei, A Danner, et al. Broadband spin-controlled focusing via logarithmic-spiral nanoslits of varying width. Laser Photonics Rev, 9, 674-681(2015).
[51] MY Li, WL Li, HY Li, YC Zhu, YT Yu. Controllable design of super-oscillatory lenses with multiple sub-diffraction-limit foci. Sci Rep, 7, 1335(2017).
[52] GY Cao, XS Gan, H Lin, BH Jia. An accurate design of graphene oxide ultrathin flat lens based on Rayleigh-Sommerfeld theory. Opto-Electron Adv, 1, 180012(2018).
[53] K Huang, HP Ye, JH Teng, SP Yeo, B Luk’yanchuk, et al. Optimization-free superoscillatory lens using phase and amplitude masks. Laser Photonics Rev, 8, 152-157(2014).
[54] K Huang, F Qin, H Liu, HP Ye, CW Qiu, et al. Planar diffractive lenses: fundamentals, functionalities, and applications. Adv Mater, 30, 1704556(2018).
[55] A Arbabi, Y Horie, AJ Ball, M Bagheri, A Faraon. Subwavelength-thick lenses with high numerical apertures and large efficiency based on high-contrast transmitarrays. Nat Commun, 6, 7069(2015).
[56] XP Li, HR Ren, X Chen, J Liu, Q Li, et al. Athermally photoreduced graphene oxides for three-dimensional holographic images. Nat Commun, 6, 6984(2015).
[57] XY Weng, LP Du, P Shi, XC Yuan. Tunable optical cage array generated by Dammann vector beam. Opt Express, 25, 9039-9048(2017).
[58] SH Xu, YM Li, LR Lou. Axial optical trapping forces on two particles trapped simultaneously by optical tweezers. Appl Opt, 44, 2667-2672(2005).
[59] YQ Zhao, QW Zhan, YL Zhang, YP Li. Creation of a three-dimensional optical chain for controllable particle delivery. Opt Lett, 30, 848-850(2005).
[60] XR Zheng, H Lin, TS Yang, BH Jia. Laser trimming of graphene oxide for functional photonic applications. J Phys D Appl Phys, 50, 074003(2017).
[61] YB Ma, GH Rui, B Gu, YP Cui. Trapping and manipulation of nanoparticles using multifocal optical vortex metalens. Sci Rep, 7, 14611(2017).
[62] Jr Hummers, RE Offeman. Preparation of graphitic oxide. J Amer Chem Soc, 80, 1339(1958).
[63] SP Boyd, L Vandenberghe. Convex Optimization(2004).
[64] M Gu. Advanced Optical Imaging Theory(2000).
[65] HP Ye, CW Qiu, K Huang, JH Teng, B Luk’yanchuk, et al. Creation of a longitudinally polarized subwavelength hotspot with an ultra-thin planar lens: vectorial Rayleigh-Sommerfeld method. Laser Phys Lett, 10, 065004(2013).
Acknowledgements
Author contributions
Competing interests
Supplementary information
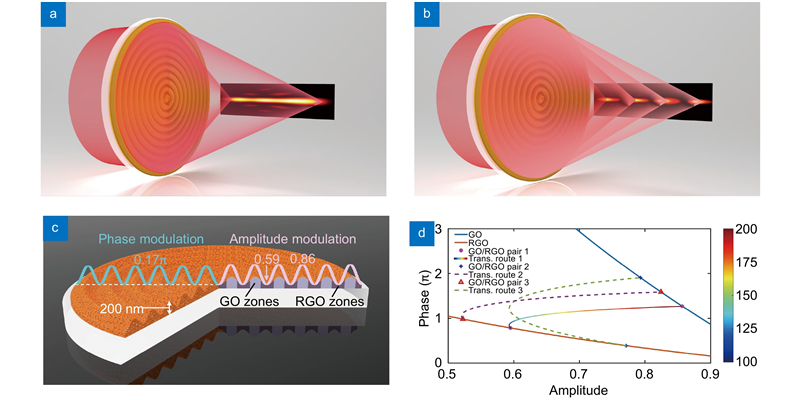
Set citation alerts for the article
Please enter your email address