Inki Kim, Juyoung Yun, Trevon Badloe, Hyuk Park, Taewon Seo, Younghwan Yang, Juhoon Kim, Yoonyoung Chung, Junsuk Rho, "Structural color switching with a doped indium-gallium-zinc-oxide semiconductor," Photonics Res. 8, 1409 (2020)

Search by keywords or author
- Photonics Research
- Vol. 8, Issue 9, 1409 (2020)
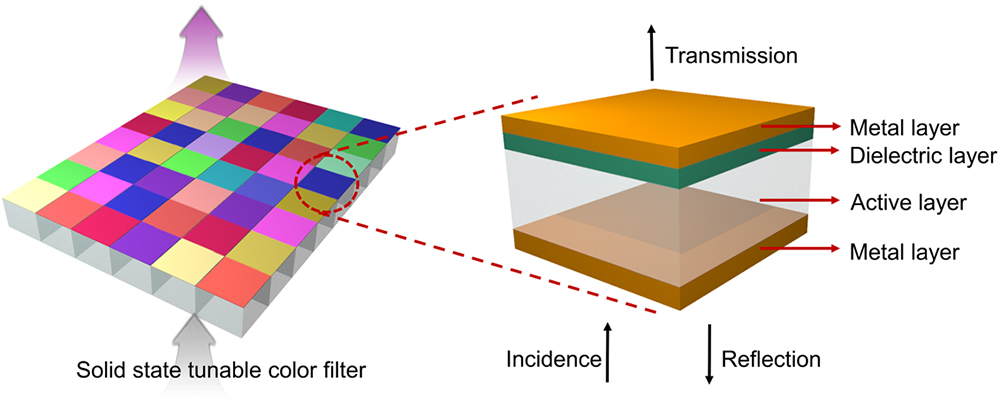
Fig. 1. Schematic of the tunable all-solid-state color filter based on a doped semiconductor. The top and bottom metal layers are silver (Ag), the passive index-changing layer is IGZO, and the dielectric spacer layer is silicon dioxide (SiO 2 ).
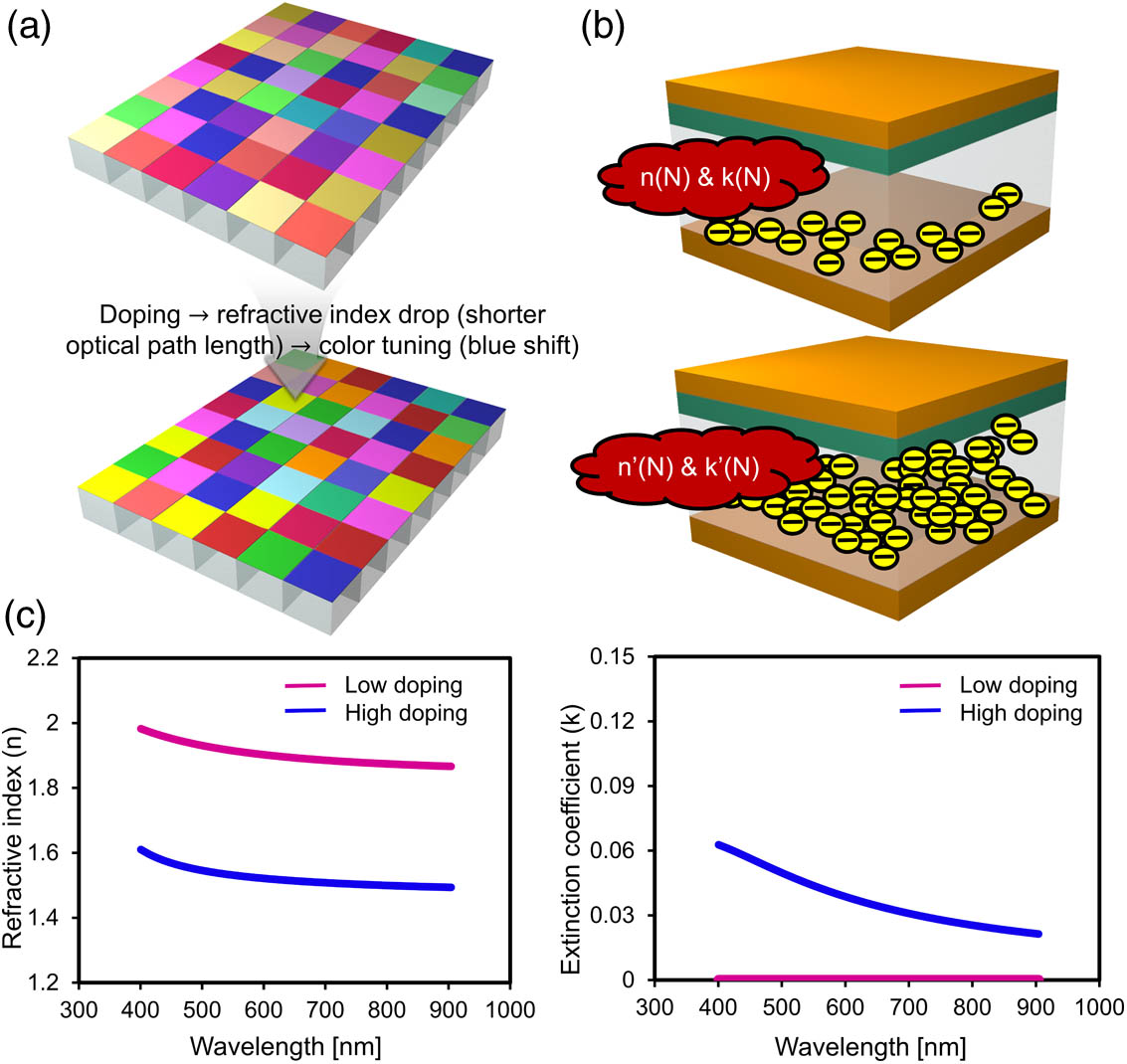
Fig. 2. Working mechanism of tunable color filter. (a) Schematic of tunable color filters. (b) The electron density and refractive index of the IGZO layer are changed under the H 2 treatment process. The thickness of the semiconducting layer can be a few tens of nanometers. (c) Corresponding modulation of the refractive index and extinction coefficient depending on the electron density. The low conductivity film had an electron density of less than 10 14 cm − 3 , and the high conductivity film had an electron density of 8 × 10 19 cm − 3 . Both samples were made of identical materials, and only the electron density was controlled.
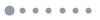
Fig. 3. FDTD simulation results. (a) Simulation result of FP type transmission color filter. Top and bottom layers use Ag as a reflecting mirror. IGZO and SiO 2 work as the dielectric spacing layer. IGZO is a transparent oxide layer and by a H 2 treatment process, the charge concentration can be tuned. The transmitted colors are obtained by varying the thickness of the SiO 2 layer. (The thickness increases from 40 nm to 180 nm in 20 nm steps.) (b) Color tuning before and after H 2 treatment. (c), (d) Simulated transmission spectra are presented where the SiO 2 layer thickness is (c) 60 nm and (d) 80 nm. (e), (f) Transmission spectrum plot as a function of wavelength and spacer thickness (e) before and (f) after H 2 treatment.
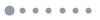
Fig. 4. Experimental results of centimeter-scale device. (a) Photograph of fabricated devices. The top row is the devices before H 2 treatment, and the bottom row is the carrier-injected devices. The increase in carrier concentration can blue shift the wavelength peak of transmitted light resulting in color change from green to blue, for example. From left to right, the SiO 2 layer increases from 40 nm to 120 nm. By increasing the thickness of the dielectric spacer, the wavelength is red shifted. The experimental results are well matched to the simulation results. Each sample size is 2 cm × 2 cm . (b) Measured transmission spectrum from non-doped devices depending on the SiO 2 layer thickness. (c) Color change map due to H 2 treatment. The dielectric layer thickness of the sample moving from green to blue is 60 nm, and the purple to brown is 120 nm. The white triangle represents sRGB color space. (d) Measured transmission spectrum with SiO 2 thickness of 120 nm.
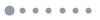
Fig. 5. Experimental results of micro-sized color printing. The device consists of four layers including top and bottom layers of Ag surrounding IGZO and SiO 2 layers. The color pixels were fabricated by an FIB process after the deposition of a 180 nm thick SiO 2 layer. (a) Optical microscope images of 20 μm sized color pixels. (b) Colorful pictures with 1 μm sized color pixels. Left: low doping IGZO; right: high doping IGZO.
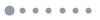
Fig. 6. Atomic force microscope (AFM) image. To characterize the fabricated micro-scale color pixel of a colorful picture, an AFM measurement was conducted. The 1 μm × 1 μm sized pixel has different depths depending on the encoded color information. (a) Top view of AFM image. The depth is described as brightness value. (b) 3D AFM image. (c) Cross-section depth information of red-dashed line in (a). The y axis of the plot represents relative positions.
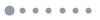
Fig. 7. Refractive index modulation. Measured refractive index of IGZO film after different H 2 plasma treatment conditions. Particularly, by changing the process temperature, the refractive index can be modulated. In this study, the H 2 plasma treatment was performed at 120°C by a plasma-enhanced chemical vapor deposition chamber.
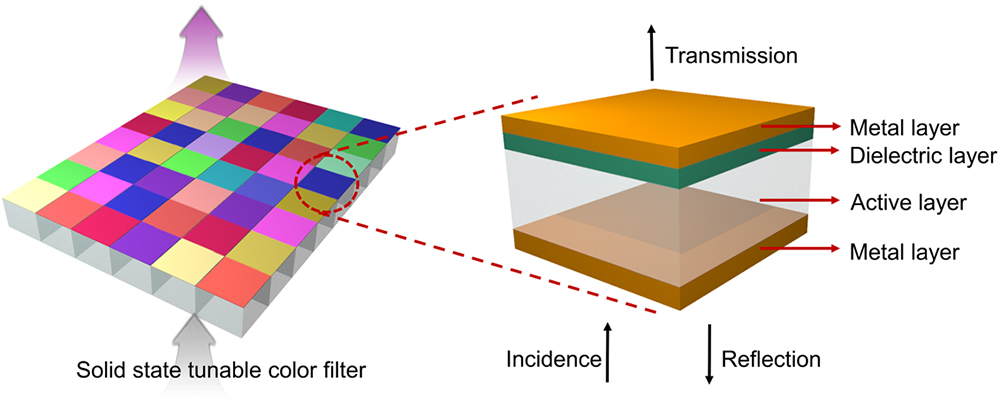
Set citation alerts for the article
Please enter your email address