Fig. 1. Schematic diagram of the indirect chiral coupling, with the resonator as a coupling interface between a point source and a waveguide. (a) Original problem under excitation by a point source at r0, with β+,m and β−,m denoting the coefficients of the excited normalized mth waveguide modes ψ+,m and ψ−,m propagating in positive and negative z directions, respectively. (b) Reciprocal problem under excitation by the ψ+,m and ψ−,m with unitary coefficient, with E+,mcoupling(r0) or E−,mcoupling(r0) denoting the excited electric field at r0, respectively. For the example of the system sketched in the figure, the source is right-handed circularly polarized (as sketched by the arrowed circle), and the structure is symmetric with respect to the z=z0 plane where the source is located. In the following figures, the ψ+,m and ψ−,m are omitted for simplicity, with only the coefficients β± being labeled.
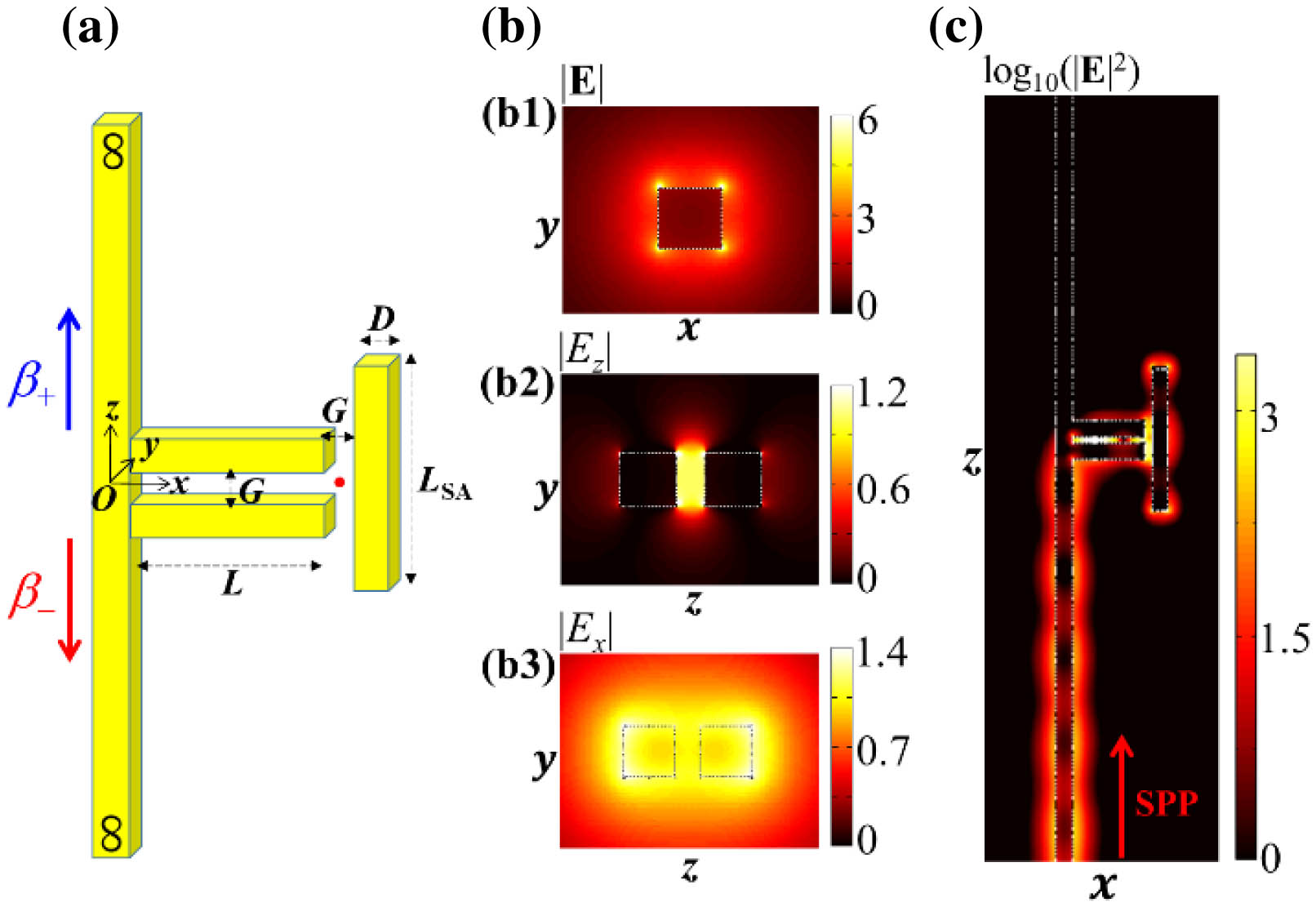
Fig. 2. Indirect chiral-coupling system between a chiral point source and the SPP waveguide mode mediated by an FP nanocavity. (a) Sketch of system. The circularly polarized point source (shown by the red dot) is located in the air gap between the SA and the right terminal of the DA. The coordinate origin O is set at the inner center of the waveguide. (b) Electric field of the fundamental SPP modes, calculated with the fully vectorial a-FMM. (b1) Distribution (in the x−y plane) of the electric-field modulus |E|=|Ex|2+|Ey|2+|Ez|2 of the fundamental SPP mode on the waveguide and SA, which satisfies the normalization of Ez=1 at the center of the waveguide and has a complex effective index of neff=1.700+0.05136i. (b2) Distribution (in the y−z plane) of the electric-field z component modulus |Ez| of the fundamental antisymmetric SPP mode on the DA, which satisfies the normalization of Ez=1 at the center of the DA and has a complex effective index of neffasym=2.423+0.07471i. (b3) Distribution of |Ex| of the fundamental symmetric SPP mode on the DA, which satisfies the normalization of Ex=1 at the center of the DA and has a complex effective index of neffsym=1.475+0.03732i. (c) Distribution (in the plane y=0) of |E|2 excited by the normalized up-going fundamental SPP on the waveguide (shown by the red arrow). The length of the DA is L=200 nm and the results are obtained with the a-FMM. The superimposed dotted lines in (b) and (c) represent the boundaries of structures.
Fig. 3. Definitions of the SPP scattering coefficients and the unknown SPP mode coefficients in the SPP model. The superscripts “sym” and “asym,” which correspond to the symmetric and antisymmetric SPPs on the DA, respectively, are omitted in the figure. (a) SPP model for the reciprocal problem. (a1) Unknown SPP mode coefficients a± and b± to be solved in the model. (a2)–(a4) Definitions of SPP scattering coefficients α±, ra, and rb, and definition of the coupling field ψR. In (a1) and (a2), the blue and red arrows or coefficients correspond to the incident SPPs on the upper and lower waveguide arms, respectively. (b) SPP model for the original problem (red dot representing the point source). (b1) Unknown SPP mode coefficients as, bs, β+, and β− to be solved in the model. (b2) Definitions of SPP excitation coefficient γ and field ψsource excited by the point source. (b3) Definition of SPP coupling coefficients χ±.
![Calculation results of the indirect chiral coupling. (a1) Coupling rates ΓSPP,± of up-going and down-going SPPs on the waveguide excited by a right-handed circularly polarized point source plotted as functions of the length L of the DA, normalized by the emission rate Γair of the source in air. The solid curves and the circles represent the results obtained with the SPP model for the reciprocal problem [Eq. (14)] and the fully vectorial a-FMM, respectively. The blue and red curves correspond to the up-going and down-going SPPs on the waveguide, respectively. The point source is located at r0=(D/2+L+7 nm, 0, 0). (a2), (a3) Moduli |aasym| and |asym| of the coefficients of the right-going antisymmetric and symmetric SPPs on the DA excited by the up-going (or down-going) SPP on the waveguide, which are obtained with the model Eq. (11a) [or Eq. (12a)]. (b) Phase difference φ of the two orthogonal components of the electric field at the position of the point source. The field is excited by an incident up-going SPP on the waveguide and is obtained with the model Eq. (17). (c) Directivity factor g obtained with the a-FMM (circles) and the SPP model (solid curve), respectively. In (a)–(c), the green vertical dashed lines from left to right correspond to n=0–6 in Eq. (16). (d) Distribution (in y=0 plane) of S3 of the electric field in the coupling region between the DA and the SA. The field is excited by an incident up-going SPP on the waveguide and is obtained with the model Eq. (13a). (e) Distribution (in y=0 plane) of the normalized electric-field intensity (|E|/Γair)2 excited by the right-handed circularly polarized point source at r0. The result is obtained with the FEM. In (d) and (e), the length of DA is L=185, 342, 500 nm from left to right, corresponding to n=1, 2, 3 in (a), respectively.](/Images/icon/loading.gif)
Fig. 4. Calculation results of the indirect chiral coupling. (a1) Coupling rates ΓSPP,± of up-going and down-going SPPs on the waveguide excited by a right-handed circularly polarized point source plotted as functions of the length L of the DA, normalized by the emission rate Γair of the source in air. The solid curves and the circles represent the results obtained with the SPP model for the reciprocal problem [Eq. (14)] and the fully vectorial a-FMM, respectively. The blue and red curves correspond to the up-going and down-going SPPs on the waveguide, respectively. The point source is located at r0=(D/2+L+7 nm, 0, 0). (a2), (a3) Moduli |aasym| and |asym| of the coefficients of the right-going antisymmetric and symmetric SPPs on the DA excited by the up-going (or down-going) SPP on the waveguide, which are obtained with the model Eq. (11a) [or Eq. (12a)]. (b) Phase difference φ of the two orthogonal components of the electric field at the position of the point source. The field is excited by an incident up-going SPP on the waveguide and is obtained with the model Eq. (17). (c) Directivity factor g obtained with the a-FMM (circles) and the SPP model (solid curve), respectively. In (a)–(c), the green vertical dashed lines from left to right correspond to n=0–6 in Eq. (16). (d) Distribution (in y=0 plane) of S3 of the electric field in the coupling region between the DA and the SA. The field is excited by an incident up-going SPP on the waveguide and is obtained with the model Eq. (13a). (e) Distribution (in y=0 plane) of the normalized electric-field intensity (|E|/Γair)2 excited by the right-handed circularly polarized point source at r0. The result is obtained with the FEM. In (d) and (e), the length of DA is L=185, 342, 500 nm from left to right, corresponding to n=1, 2, 3 in (a), respectively.
Fig. 5. Distribution (in y=0 plane) of the normalized electric-field intensity (|E|/Γair)2 excited by a point source at r0 which is (a) left-handed circularly polarized, (b) linearly polarized in direction of 45° from the x axis, (c) right-handed elliptically polarized [p=x(3−1)/2+iz(3+1)/2], or (d) left-handed elliptically polarized [p=x(3−1)/2−iz(3+1)/2]. In (a)–(d), the length of DA is L=185 nm, corresponding to n=1 in Eq. (16). The results are obtained with the FEM.
Fig. 6. Purcell factor FP=Γtotal/Γair of the chiral source and coupling efficiency η± of the SPP waveguide mode. The solid curves and the circles represent the results obtained with the SPP model for the original problem and the fully vectorial a-FMM, respectively. The green vertical dashed lines from left to right correspond to n=0–6 in Eq. (16). (a) Results of the Purcell factor Γtotal/Γair of a chiral point source of right-handed circular polarization at r0=(D/2+L+7 nm, 0, 0). (b) Results of the coupling efficiency η±=ΓSPP,±/Γtotal of the up-going (blue curve) and down-going (red curve) SPPs on the waveguide excited by the chiral point source at r0.
Fig. 7. Integral path (blue lines) and poles (red dots) of the integrand for the integration in Eq. (A8). (a) and (b) are for the cases of z−z0>0 and z−z0<0, respectively. For z−z0>0 (z−z0<0), the closed integral path is composed of the real axis and a semi-circle C+ (C−) in the upper (lower) complex plane with an infinite radius R, in which the integrand f(k) has a single first-order pole of km (−km).
![Numerical example showing the higher accuracy of Eq. (A17) (our theory) compared with Eq. (A19) (previous theories) for lossy waveguide modes. (a) Sketch of the direct chiral-coupling system composed of a gold-nanowire waveguide and a nearby chiral source (the red dot). The waveguide is identical to that considered in Fig. 2(b1) in the main text and supports the fundamental lossy SPP mode as shown in Fig. 2(b1). A right-handed circularly polarized point source is located at r0=(x0,y0,z0)=(D/2+15 nm,0,0), with the coordinate origin O set at the inner center of the waveguide. (b) Relative error of Ez,+(0) of the excited forward-propagating SPP waveguide mode (see its detailed definition in the text) obtained with Eq. (A17) (blue solid curve) and Eq. (A19) (blue dashed curve) for different wavelengths. The inset shows the imaginary part of the complex effective index neff of the SPP waveguide mode plotted as a function of the wavelength. In calculating the Ez,+(0) with Eq. (A17), Eq. (A19), and the mode-orthogonality theorem, the full-wave aperiodic Fourier modal method (a-FMM) [74,75] is used to obtain the fundamental SPP waveguide mode and the total electromagnetic field excited by the point source.](/Images/icon/loading.gif)
Fig. 8. Numerical example showing the higher accuracy of Eq. (
A17) (our theory) compared with Eq. (
A19) (previous theories) for lossy waveguide modes. (a) Sketch of the direct chiral-coupling system composed of a gold-nanowire waveguide and a nearby chiral source (the red dot). The waveguide is identical to that considered in Fig.
2(b1) in the main text and supports the fundamental lossy SPP mode as shown in Fig.
2(b1). A right-handed circularly polarized point source is located at
r0=(x0,y0,z0)=(D/2+15 nm,0,0), with the coordinate origin
O set at the inner center of the waveguide. (b) Relative error of
Ez,+(0) of the excited forward-propagating SPP waveguide mode (see its detailed definition in the text) obtained with Eq. (
A17) (blue solid curve) and Eq. (
A19) (blue dashed curve) for different wavelengths. The inset shows the imaginary part of the complex effective index
neff of the SPP waveguide mode plotted as a function of the wavelength. In calculating the
Ez,+(0) with Eq. (
A17), Eq. (
A19), and the mode-orthogonality theorem, the full-wave aperiodic Fourier modal method (a-FMM) [
74,
75] is used to obtain the fundamental SPP waveguide mode and the total electromagnetic field excited by the point source.
Fig. 9. (a) Total spontaneous emission rate Γtotal of an x-polarized point source plotted as a function of the length LSA of the SA. Γtotal is normalized by the spontaneous emission rate Γair of the point source in air, with Γair=ηvack02na/(12π). The structure and coordinate system are shown in the inset, with coordinate origin O at the inner center of the antenna. The coordinate of the point source (shown by the red dot) is (x,y,z)=(−D/2−13 nm,0,0), with D=40 nm being the side length of the antenna cross section. (b) Distribution of normalized electric-field intensity (|E|/Γair)2 excited by the point source, obtained for LSA=370 nm and in sections y=0 (left) and x=0 (right).
Fig. 10. Distribution (in y=0 plane) of the normalized electric-field intensity (|E|/Γair)2 in the structure without the SA (a1) or without the DA and the SA (a2), which is excited by a right-handed circularly polarized point source at r0 corresponding to a DA length of L=185 nm [i.e., n=1 in Eq. (16)]. (b1), (b2) The same as (a1) and (a2) but for L=500 nm [i.e., n=3 in Eq. (16)]. The results are obtained with the FEM.
Fig. 11. (a) Sketch of the direct chiral-coupling system, which is composed of a bare SPP waveguide excited by a nearby right-handed circularly polarized point source (shown by the red dot). The waveguide is a gold nanowire with a square cross section of side length D=40 nm (identical to the waveguide in the indirect chiral-coupling system proposed in the main text). The point source is located at r0=(D/2+d, 0, 0), with the coordinate origin O set at the inner center of the waveguide. (b) Purcell factor FP=Γtotal/Γair of the point source plotted as a function of the source-waveguide distance d. (c) Coupling efficiency η±=ΓSPP,±/Γtotal of the up-going (blue curve) and down-going (red curve) fundamental SPP modes on the waveguide, and the directivity factor g (green curve) plotted as functions of d. The results are obtained with the full-wave a-FMM.
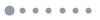
Fig. 12. (a) Sketch of the indirect chiral-coupling system on a glass substrate in air. The gold nanowires have a square cross section with a side length D=40 nm, and the gaps in the DA and between the DA and SA are all of width G=20 nm, which are identical to those of the system without a substrate. The coordinate origin O is set at the inner center of the waveguide. The right-handed circularly polarized point source is located at r0=(D/2+L+7 nm, 15 nm, 0), with L being the length of the DA. The length of the SA is LSA=290 nm. (b) Coupling rates ΓSPP,± of up-going (blue curve) and down-going (red curve) fundamental SPP modes on the waveguide excited by the point source, which are plotted as functions of L and normalized by the emission rate Γair of the source in air. (c) Directivity factor g of the excited SPPs. (d) Purcell factor FP=Γtotal/Γair of the point source. (e) Coupling efficiency η±=ΓSPP,±/Γtotal of the up-going (blue curve) and down-going (red curve) SPPs. In (b)–(e), the results are obtained with the full-wave FEM. The vertical green-dashed lines show the values of L determined by the phase-matching condition of Eq. (16) in the main text.