Abstract
ZnO films containing Er and Ge nanocrystals (nc-Ge) were synthesized and their photoluminescence (PL) properties were studied. Visible and near-infrared PL intensities are found to be greatly increased in nc-Ge-containing film. Er-related 1.54 μm emission has been investigated under several excitation conditions upon different kinds of Ge, Er codoped ZnO thin films. 1.54 μm PL enhancement accompanied by the appearance of nc-Ge implies a significant correlation between nc-Ge and PL emission of Er3+. The increased intensity of 1.54 μm in Ge:Er:ZnO film is considered to come from the joint effect of the local potential distortion around Er3+ and the possible energy transfer from nc-Ge to Er3+.1. INTRODUCTION
Doping erbium into semiconductors has attracted enormous interest in optical applications as Er-related 1.54 μm emission is within a wavelength range of minimum loss for silica optical fibers [1]. It has been reported that oxygen codoping and the use of wide-bandgap materials as the host material are effective to enhance the Er-related 1.54 μm emission [2,3]. ZnO is considered to be a promising candidate as a host material for Er doping because of being an oxide with a wide bandgap of about 3.37 eV [4,5]. However, the efficiency of 1.54 μm emission in ZnO:Er thin films has not yet been satisfied. It has been confirmed that through the addition of Si nanocrystals (nc-Si) into , strong photoluminescence (PL) at room temperature could be obtained [6–10]. Ge nanocrystals (nc-Ge) have many properties (wide size-dependent emission tunability, larger Bohr radius, etc.), which is superior to nc-Si [11]. The luminescence efficiency of may be enhanced by the introduction of nc-Ge through the change of local environment and recombination of photogenerated excitons in nanocrystals and subsequent energy transfer to .
Energy transfer from nc-Si to in a silica host has been intensively investigated in recent years. In comparison, few studies have been found on nc-Ge and in a ZnO host. In this paper, we deposit ZnO film with codoped Ge, Er in it by magnetron sputtering. Visible and near-infrared (NIR) PL intensities are found to be greatly increased in 600°C annealed Ge:Er:ZnO film compared to the as-deposited sample and the sample without Ge dopants. A 1.54 μm PL enhancement accompanied by the appearance of nc-Ge in an annealed sample implies a significant correlation between nc-Ge and . The purpose of this work is to analyze how the nc-Ge participates in the PL enhancement. Our observed results suggest that the increased intensity of 1.54 μm in Ge:Er:ZnO film is the result of the joint effect of two factors: local distortion effect and the energy transfer from the nc-Ge participating in the efficient emission.
A ( at. %) target with small pieces of Ge chips was cosputtered by radio frequency magnetron sputtering onto substrates. In the current experiment, Ge concentration was fixed to be about 4% in volume fraction. The deposition was carried out in an oxygen and argon mixed gas atmosphere (), and the chamber pressure was maintained at a constant value of 0.7 Pa. After deposition, the Ge:Er:ZnO film thickness was measured to be about 400 nm. Postannealing of the film was performed for 20 min at temperature of 600°C in atmosphere. As a comparison, the Ge:Er:ZnO film ( at. %) and the Er:ZnO film ( at. %) were also deposited under the same experimental conditions. PL measurements were performed at room temperature using the 325 and 532 nm lasers, and a photomultiplier tube and a thermo-electrically cooled InGaAs detector were used to detect the visible and infrared PL signals, respectively. PL excitation (PLE) spectra measurements are carried out by excitation from the wide spectrum of a xenon lamp.
Sign up for Photonics Research TOC. Get the latest issue of Photonics Research delivered right to you!Sign up now
2. MATERIALS AND METHODS
Crystalline Ge particles in nanometer size are formed by the annealing process. Figure 1(a) shows a typical cross-section high resolution transmission electron microscopy (HRTEM) image of the 600°C annealed Ge:Er:ZnO ( at. %) film containing nc-Ge. The lattice fringes correspond to the (111) planes of Ge crystals, where the diamond structure can be seen. The fast Fourier transform process in Fig. 1(a) is carried out to distinguish nc-Ge and ZnO; it is shown that nc-Ge is formed in the square lattice while that of ZnO is the hexagonal structure. These nc-Ge distribute dispersedly in ZnO matrices, and the estimated average diameter of them is about 5 nm. The morphology and distribution of Ge analyzed by high angle annular dark-field imaging (HAADF), as shown in Fig. 1(b), match the results of HRTEM well.
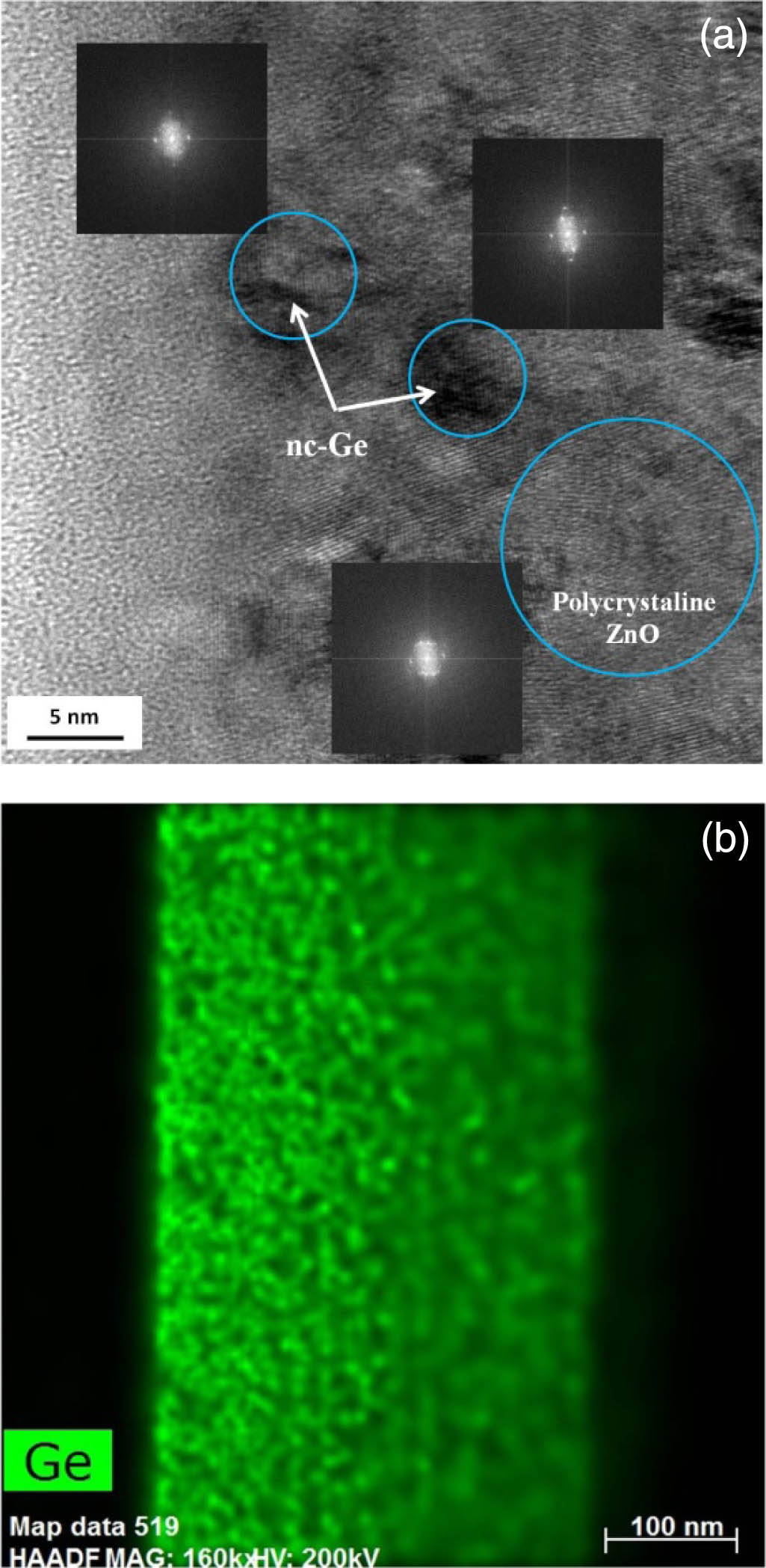
Figure 1.(a) Cross-section HRTEM image of well-grown nc-Ge 5 nm in size in 600°C annealed Ge:Er:ZnO ( at. %) films. (b) HAADF image of the above film.
3. RESULTS AND DISCUSSION
Figure 2 shows the visible PL spectra from as-deposited, 600°C annealed Ge:Er:ZnO and 600°C annealed Er:ZnO films (all with at. %) under excitation at 325 nm. A broad luminescence with a peak at about 0.58 μm can only be found in the 600°C annealed Ge:Er:ZnO sample. The visible luminescence appears accompanied by the appearance of nc-Ge. It may come from different emission sources, such as new defect states in ZnO originated from the incorporation of nc-Ge [12] and the recombination of photogenerated excitons in nc-Ge due to their quantum confinement effect [13,14]. About the contribution from the latter, a zeroth-order approximation asymptotic wave function is used to calculate the possible PL emission of nc-Ge [14,15]. The energy (the emission wavelength peak equal to 0.569 μm) can be obtained, where the radius of nc-Ge, , is employed according to our HRTEM result. This value is in good consistent with the visible PL result shown in Fig. 2.
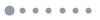
Figure 2.Visible PL spectra of as-deposited, 600°C annealed Ge:Er:ZnO and 600°C annealed Er:ZnO films (all with at. %).
To analyze the NIR PL of ions in Ge:Er:ZnO, three possible excitation approaches are investigated: energy transfer from the 3.37 eV band-edge excitation of the ZnO host [16], direct excitation of the main absorption levels of by the pump light, and indirect excitation through energy transfer from photogenerated excitons in nc-Ge to . Figure 3 shows the NIR PL spectra of the as-deposited, 600°C annealed Ge:Er:ZnO and 600°C annealed Er:ZnO films (all with at. %) under excitations at 325 and 532 nm. PL peaks at 0.998 and 1.54 μm correspond to the intra-4f transition of and to the ground state , respectively [17]. Pumping light at 325 nm corresponds to the band-tail absorption of the ZnO host, and 1.54 μm emission can be achieved through an indirect excitation by energy transfer from recombination of electron–hole () pairs in the ZnO host to ions. From Fig. 3(a), it can be seen that 1.54 μm emission of the samples without nc-Ge (as-deposited Ge:Er:ZnO and Er:ZnO films) are very low. It indicates that the energy transfer from the ZnO band-edge transition to is extremely inefficient. However, this situation has been changed dramatically with the addition of nc-Ge: a strong PL at 1.54 μm and an intra-4f transition at 0.998 μm are observed from the 600°C annealed Ge:Er:ZnO film. It provides evidence for the enhancement effect of nc-Ge on 1.54 μm PL. Beyond the band-tail absorption of ZnO, pumping with 532 nm excludes the possibility of the energy transfer mechanism through ZnO. Direct excitation of exists in all the Er:ZnO film. 532 nm light directly excites ions from ground state to higher-energy levels at and consequently relax to to give emission at 1.54 μm. However, it is shown in Fig. 3(b) that such direct excitation is demonstrated to be extremely low without nc-Ge. Compared to the weak NIR PL from Er:ZnO and as-deposited Ge:Er:ZnO films, 1.54 μm emission from nc-Ge-containing film is greatly enhanced. This indicates that nc-Ge plays a significant role in excitation.
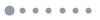
Figure 3.1.54 μm PL spectra of the as-deposited, 600°C annealed Ge:Er:ZnO and Er:ZnO films (all with at. %) under different excitation wavelengths. The arrows denoted in the diagram indicate each corresponding transition level to the ground state in ions. The inset in (a) shows the energy diagram of ions.
Optical spectra measurements are carried out to analyze how the nc-Ge participates in the PL enhancement. Figure 4(a) shows the PLE spectrum monitored at 1.54 μm as a function of the excitation wavelength in the 300–750 nm range for Er:ZnO without Ge and nc-Ge-containing Er:ZnO films. It can be found that compared to the sample without Ge dopants, both the energy transfer from the ZnO host and direct excitation of are more efficient in nc-Ge-containing film, and the overall luminous efficiency is much higher in it. Meanwhile, the PLE peak of the nc-Ge-containing Er:ZnO film shifts to short wavelengths. This indicates that the local environment of 4f states of Er ions has been modified by the formation of nc-Ge. It has been reported that lowering of the symmetry of the crystal field around is more suitable for Er intra-4f transitions [18,19]. In order to achieve such local potential distortion of the optically active centers, doping some impurities into the specimen proves to be very effective. The existence of nc-Ge may strongly affect the local environment of Er, which enhances the transition rates, resulting in a large enhancement of the 1.54 μm PL intensity. In addition, energy transfer from nc-Ge to may be another possible reason for PL enhancement. Figure 4(b) shows the dependence of visible spectra for 600°C annealed nc-Ge-containing ZnO films with different Er concentrations (0.6 at. % and 0.3 at. %) under excitation at 325 nm. If the energy transfer from nc-Ge to is assumed, the emission intensities between yellow band emission and Er-related 1.54 μm emission may correlate to each other depending on the number of surrounding the nc-Ge. In Fig. 4(b), it can be found that the visible PL decreases and NIR PL at 1.54 μm increases as the Er concentration increases. According to semi-quantum theory, when the quantum dot size of nc-Ge is smaller than its effective Bohr radius (24 nm for Ge), the carrier (electrons, holes) movement will be limited, leading to the increase of kinetic energy and the discrete of energy levels in nc-Ge [20]. The quantum confinement effect of nc-Ge encourages the excitation at (0.52 μm in Fig. 2; 0.52 and 0.58 μm may be the most efficient energy transfer wavelength and the nc-Ge luminescence wavelength, respectively), and this energy is resonant with the transition of [inset of Fig. 3(a)]. The resonant energy transfer will make 1.54 μm emission more efficient. Although the PLE spectra do not reflect the absorption band of nc-Ge, probably due to the weak PL intensity from nc-Ge compared to that from direct excitations or there may exist a fast energy exchange between nc-Ge and nearby , the possible energy transfer from nc-Ge to cannot be ruled out. We have found that the 600°C annealed process resulted in the strongest PL intensity. If the annealing temperature is increased further, as the size of nc-Ge increases, 1.54 μm PL intensity decreases dramatically. This is probably due to the fact that with the decrease in the number of nc-Ge and the reduction of quantum effects, the energy exchange between nc-Ge and becomes less efficient. The 1.54 μm PL enhancement may come from a joint effect of both the local environment change of and the energy exchange between nc-Ge and . In order to find direct evidence of the energy transfer from nc-Ge to , a further investigation is being carried out.
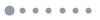
Figure 4.(a) PLE spectra observed at 1.54 μm for 600°C annealed Ge:Er:ZnO and Er:ZnO (all with at. %) films. Arrows in the diagram indicate the different excitation ways of ions. (b) Visible and NIR PL spectra of 600°C annealed Ge:Er:ZnO films containing nc-Ge with different Er concentrations (0.6 at. % and 0.3 at. %).
4. CONCLUSIONS
In conclusion, visible and NIR luminescence properties of ZnO films containing Ge dopants and are studied under different pumping wavelengths. Nc-Ge particles in size are formed in 600°C annealed Ge:Er:ZnO film. With the presence of nc-Ge, 1.54 μm emission has been greatly enhanced. The increased intensity of 1.54 μm in Ge:Er:ZnO film is considered to be the result of the joint effect of the local potential distortion around and the energy transfer from nc-Ge to .
References
[1] Y. Yang, Y. Li, L. Xiang, X. Ma, D. Yang. Low-voltage driven ∼1.54 μm electroluminescence from erbium-doped ZnO/p+-Si heterostructured devices: energy transfer from ZnO host to erbium ions. Appl. Phys. Lett., 102, 181111(2013).
[2] R. Morea, A. Miguel, T. T. Fernandez, B. Maté, F. J. Ferrer, C. Maffiotte, J. Fernandez, R. Balda, J. Gonzalo. Er3+-doped fluorotellurite thin film glasses with improved photoluminescence emissions at 1.53 μm. J. Lumin., 170, 778-784(2016).
[3] Y. Chen, Y. Lin, Y. Zou, Z. Luo, Y. Huang. Passively Q-switched 1.5–1.6 μm Er:Yb:LuAl3(BO3)4 laser with Co2+:Mg0.4Al2.4O4 saturable absorber. Opt. Express, 20, 9940-9947(2012).
[4] A. K. Pradhan, L. Douglas, H. Mustafa, R. Mundle, D. Hunter, C. E. Bonner. Pulsed-laser deposited Er:ZnO films for 1.54 μm emission. Appl. Phys. Lett., 90, 072108(2007).
[5] L. Honglin, L. Yingbo, L. Jinzhu, Y. Ke. Experimental and first-principles studies of structural and optical properties of rare earth (RE = La, Er, Nd) doped ZnO. J. Alloys Comp., 617, 102-107(2014).
[6] H. Krzyzanowska, Y. Fu, K. S. Ni, P. M. Fauchet. Efficient energy transfer between Si nanostructures and Er located at a controlled distance. ACS Photon., 3, 564-570(2016).
[7] N. Fukata, H. Morihiro, R. Shirakawa, K. Murakami, M. Mitome, Y. Bando. Formation of Si nanocrystallites observed by in situ transmission electron microscopy and their effect on the enhancement of Er photoluminescence in Er-doped SiO2. J. Appl. Phys., 102, 114309(2007).
[8] I. Izeddin, D. Timmerman, T. Gregorkiewicz, A. S. Moskalenko, A. A. Prokofiev, I. N. Yassievich, M. Fujii. Energy transfer in Er-doped SiO2 sensitized with Si nanocrystals. Phys. Rev. B, 78, 035327(2008).
[9] L. Dal Negro, R. Li, J. Warga, S. N. Basu. Sensitized erbium emission from silicon-rich nitride/silicon superlattice structures. Appl. Phys. Lett., 92, 181105(2008).
[10] Y.-W. Lu, C. Huang, J.-G. Cheng, A. N. Larsen. High Er3+ luminescent efficiency in Er-doped SiOx films containing amorphous Si nanodots. J. Alloys Comp., 676, 428-431(2016).
[11] Z. Wang, S. Wang, Y. Yin, T. Liu, D. Lin, D. H. Li, X. Yang, Z. Jiang, Z. Zhong. Promising features of low-temperature grown Ge nanostructures on Si (001) substrates. Nanotechnology, 28, 115701(2017).
[12] A. B. Djurisic, W. C. H. Choy, V. A. L. Roy, Y. H. Leung, C. Y. Kwong, K. W. Cheah, T. K. Gundu Rao, W. K. Chan, H. F. Lui, C. Surya. Photoluminescence and electron paramagnetic resonance of ZnO tetrapod structures. Adv. Funct. Mater., 14, 856-864(2004).
[13] Y. Maeda, N. Tsukamoto, Y. Yazawa, Y. Kanemitsu, Y. Masumoto. Visible photoluminescence of Ge microcrystals embedded in SiO2 glassy matrices. Appl. Phys. Lett., 59, 3168-3170(1991).
[14] Y. Maeda. Visible photoluminescence from nanocrystallite Ge embedded in a glassy SiO2 matrix: evidence in support of the quantum-confinement mechanism. Phys. Rev. B, 51, 1658-1670(1995).
[15] L. Brus. Zero-dimensional ‘excitons’ in semiconductor clusters. IEEE J. Quantum Electron., 22, 1909-1914(1986).
[16] F. Xiao, R. Chen, Y. Q. Shen, Z. L. Dong, H. H. Wang, Q. Y. Zhang, H. D. Sun. Efficient energy transfer and enhanced infrared emission in Er-doped ZnO–SiO2 composites. J. Phys. Chem. C, 116, 13458-13462(2012).
[17] S. Komuro, T. Katsumata, T. Morikawa, X. Zhao, H. Isshiki, Y. Aoyagi. 1.54 μm emission dynamics of erbium-doped zinc-oxide thin films. Appl. Phys. Lett., 76, 3935-3937(2000).
[18] Z. Zhou, T. Komori, T. Ayukawa, H. Yukawa, M. Morinaga, A. Koizumi, Y. Takeda. Li- and Er-codoped ZnO with enhanced 1.54 μm photoemission. Appl. Phys. Lett., 87, 091109(2005).
[19] R. Zamiri, A. Rebelo, H. A. Ahangar, M. S. Belsley, J. M. F. Ferreir. Enhancement of near infrared emission in La co-doped ZnO/Er nanoplates. Ceram. Int., 40, 12947-12951(2014).
[20] T. Takagahara, K. Takeda. Theory of the quantum confinement effect on excitons in quantum dots of indirect-gap materials. Phys. Rev. B, 46, 15578-15581(1992).