Sicen Tao, Tao Hou, Yali Zeng, Guangwei Hu, Zixun Ge, Junke Liao, Shan Zhu, Tan Zhang, Cheng-Wei Qiu, Huanyang Chen, "Anisotropic Fermat’s principle for controlling hyperbolic van der Waals polaritons," Photonics Res. 10, B14 (2022)

Search by keywords or author
- Photonics Research
- Vol. 10, Issue 10, B14 (2022)
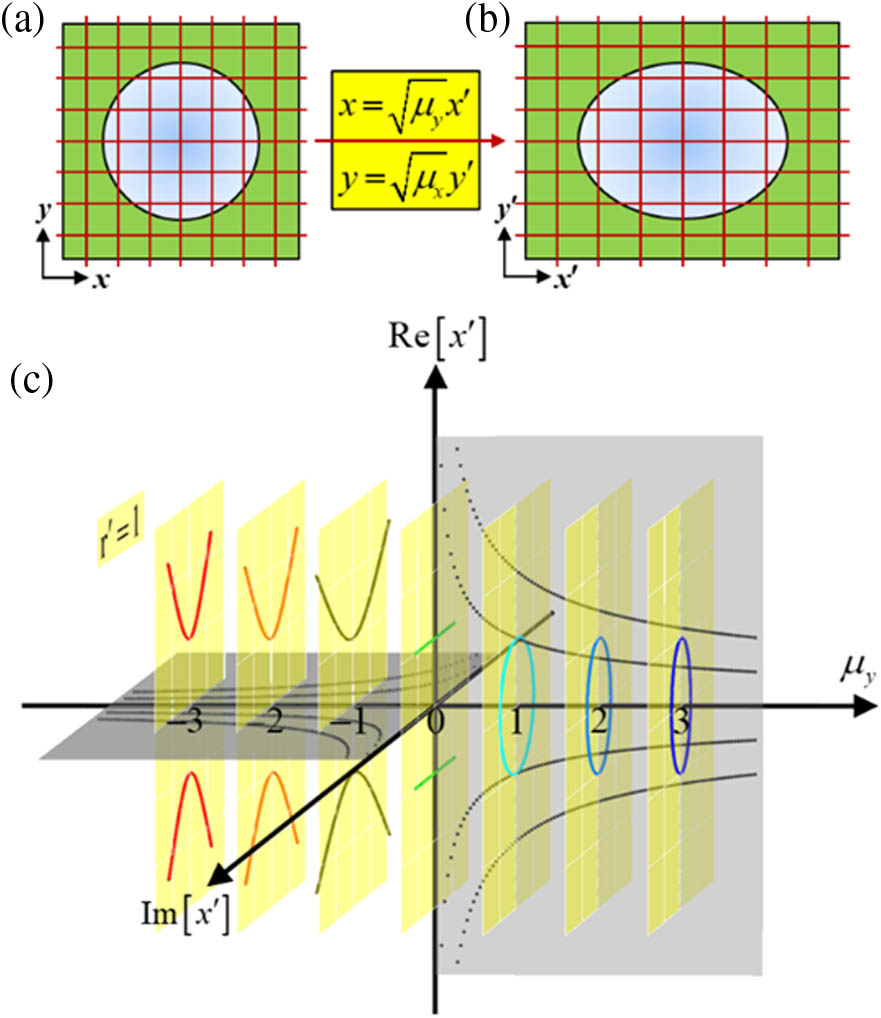
Fig. 1. Schematic of a transformation relation. (a) The original space, which corresponds to the isotropic space w = x + i y (the red square grids). (b) The physical space, which corresponds to the anisotropic space w ′ = μ y x ′ + i μ x y ′ (the red rectangular grids). (c) The transformation relations of Re ( x ′ ) − μ y with μ x = 1 and μ y ≥ 0 will be changed to Im ( x ′ ) − μ y when μ y < 0 with μ x = 1 and x = − 2 , − 1 , 1, and 2, respectively (black stipple lines). The contour curve of r ′ = 1 relevantly changes from hyperbola to parallel straight lines to an ellipse with μ y from − 3 to 0 to 3.
![Hyperbolic Luneburg lens with a collimating effect. (a), (d), (b), (e), (c), and (f), respectively, are the geometrical light behaviors, electromagnetic wave pattern [Re(Ez)], and polaritonic wave pattern [Re(Ez)] from the point source at (0,1) or (1,2).](/richHtml/prj/2022/10/10/B14/img_002.jpg)
Fig. 2. Hyperbolic Luneburg lens with a collimating effect. (a), (d), (b), (e), (c), and (f), respectively, are the geometrical light behaviors, electromagnetic wave pattern [Re ( E z ) ], and polaritonic wave pattern [Re ( E z ) ] from the point source at (0,1) or ( 1 , 2 ) .
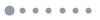
Fig. 3. (a) Schematic of the 2D model; (b) schematic of the 3D waveguide model; (c) relation between the effective refractive index n eff and the thickness d obtained from the dispersion relation Eq. (11 ).
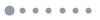
Fig. 4. Thickness distribution d ( x , y ) of (a) hyperbolic collimating LL and (b) hyperbolic focusing MFL with an aerial view (left) and top view (right).
![Hyperbolic Maxwell’s fish-eye lens with the focusing effect. (a), (d), (b), (e), (c), and (f), respectively, are the geometrical light behaviors, the electromagnetic wave pattern [Re(Ez)], and the polaritonic wave pattern [Re(Ez)] from the point source at (0, 1) or (1,2).](/Images/icon/loading.gif)
Fig. 5. Hyperbolic Maxwell’s fish-eye lens with the focusing effect. (a), (d), (b), (e), (c), and (f), respectively, are the geometrical light behaviors, the electromagnetic wave pattern [Re ( E z ) ], and the polaritonic wave pattern [Re ( E z ) ] from the point source at (0, 1) or ( 1 , 2 ) .
![Polaritonic wave pattern [Re(Ez)] of the hyperbolic Luneburg lens with a collimating effect at a frequency 634 cm−1 where the maximum thicknesses dmax of the α–MoO3 layer are (a) 220 nm, (b) 130 nm, (c) 65 nm, respectively.](/Images/icon/loading.gif)
Fig. 6. Polaritonic wave pattern [Re ( E z ) ] of the hyperbolic Luneburg lens with a collimating effect at a frequency 634 cm − 1 where the maximum thicknesses d max of the α – MoO 3 layer are (a) 220 nm, (b) 130 nm, (c) 65 nm, respectively.
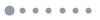
Fig. 7. Real-part permittivities of α – MoO 3 where three different Reststrahlen bands of α – MoO 3 are shaded in different colors.
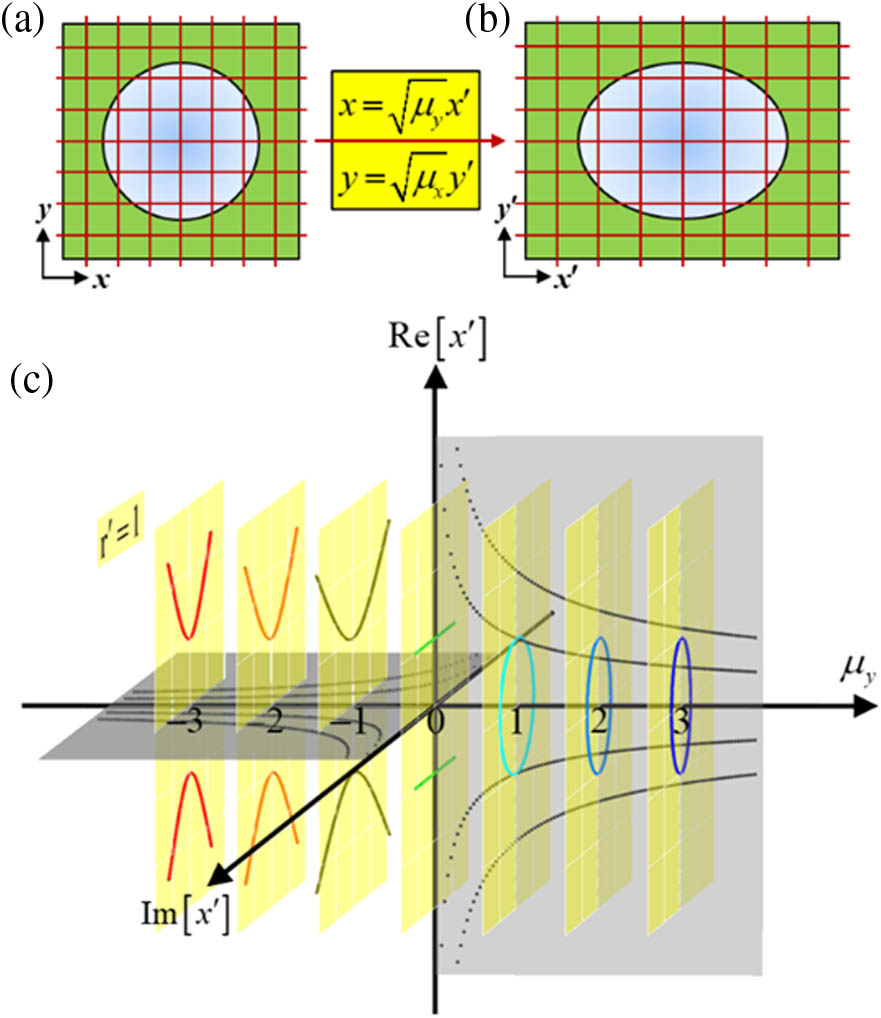
Set citation alerts for the article
Please enter your email address