Guohui Li, Rui Gao, Yue Han, Aiping Zhai, Yucheng Liu, Yue Tian, Bining Tian, Yuying Hao, Shengzhong Liu, Yucheng Wu, Yanxia Cui, "High detectivity photodetectors based on perovskite nanowires with suppressed surface defects," Photonics Res. 8, 1862 (2020)

Search by keywords or author
- Photonics Research
- Vol. 8, Issue 12, 1862 (2020)
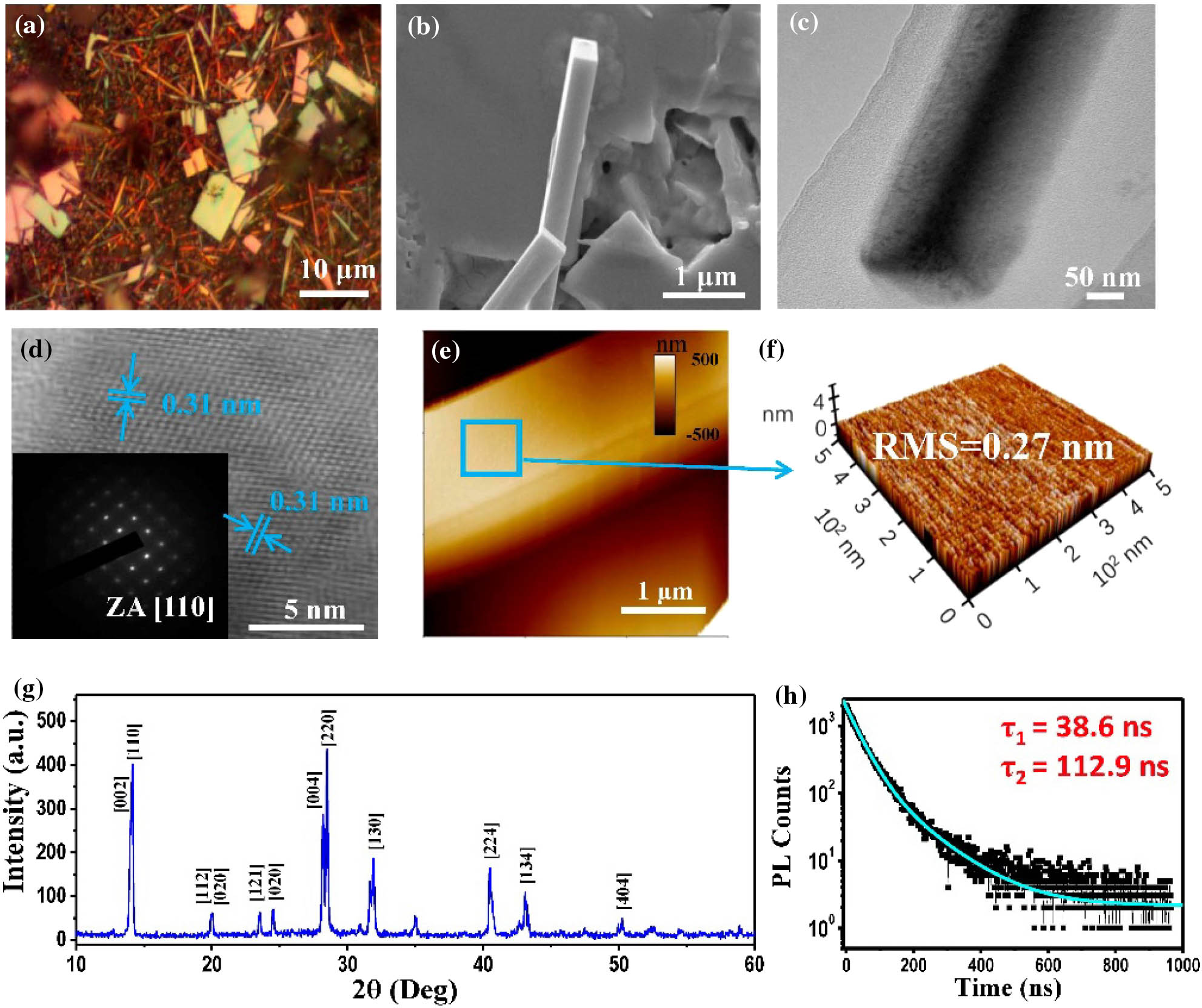
Fig. 1. (a) Optical image of MAPbI 3 nanostructures grown from PbAc 2 thin film. (b) Cross-sectional SEM image of a single MAPbI 3 nanowire. (c) Low-resolution TEM image of a single MAPbI 3 nanowire. (d) High-resolution TEM image of a selected area of a single MAPbI 3 nanowire; the inset represents its corresponding FFT pattern. (e) AFM image of a single MAPbI 3 nanowire. (f) AFM image of the selected nanowire region with the average surface roughness measured to be 0.27 nm. (g) XRD pattern of as-grown MAPbI 3 nanowires with a comparison of the simulated tetragonal phase MAPbI 3 . (h) Transient PL spectrum of the MAPbI 3 nanowire.
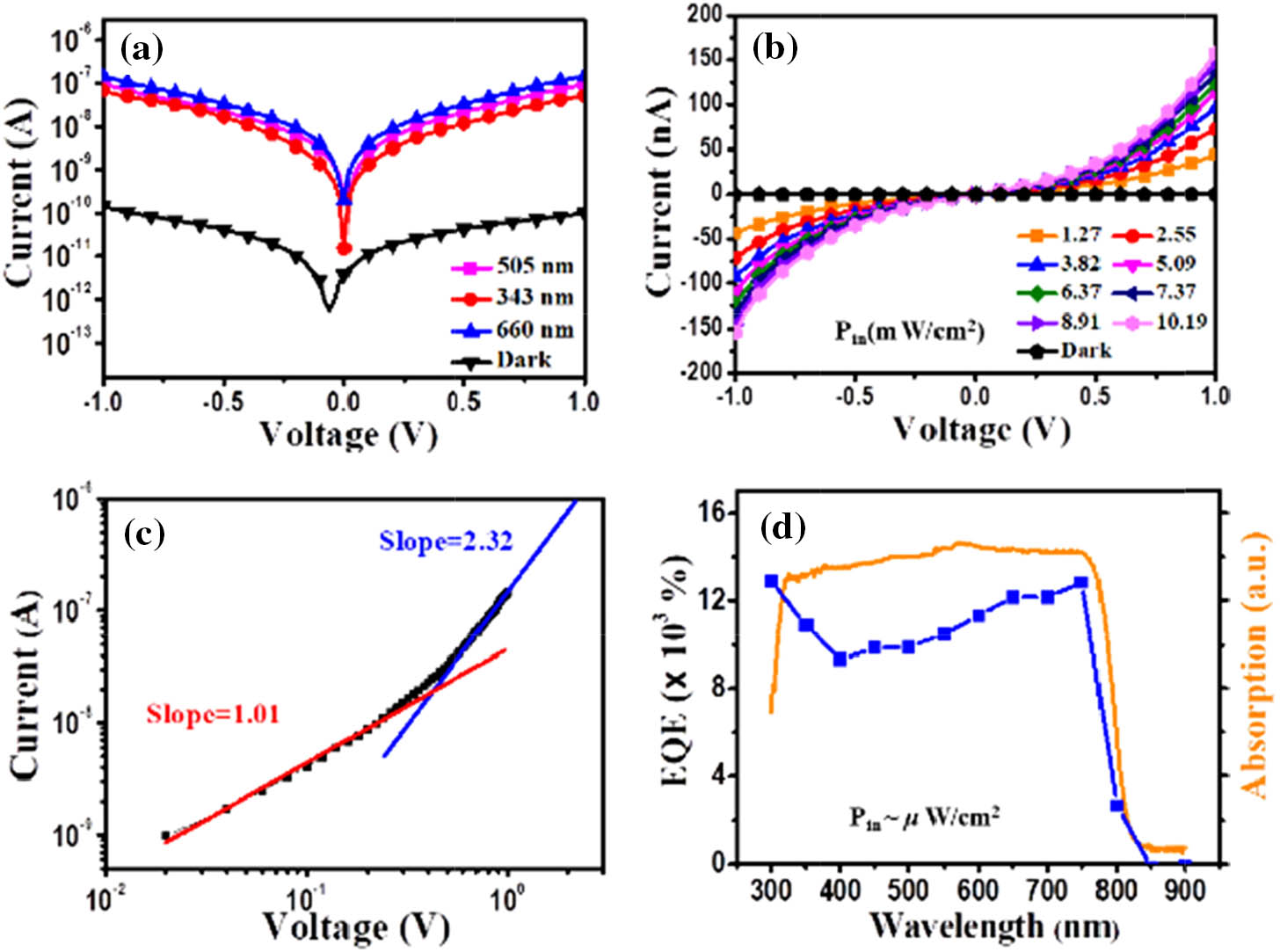
Fig. 2. (a) Logarithmic I - V curves under 325-nm, 505-nm, and 660-nm LED illumination at the power density of 10.19 mW / cm 2 . (b) I - V curves under 660-nm illumination with different power densities. (c) Double logarithmic I - V plot at the power density of 10.19 mW / cm 2 , 660 nm. (d) Wavelength-dependent EQE response and the absorption spectrum of the MAPbI 3 nanowire photodetector.
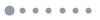
Fig. 3. (a) Current versus illumination power density (i.e., LDR measurement) of the prepared photodetector under 532-nm continuous laser illumination. (b) External quantum efficiency (EQE) versus illumination power density. (c) Responsivity (R ) versus the illumination power density. (d) Detectivity (D * ) versus illumination power density.
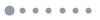
Fig. 4. (a) Transient photo response measurement of the fabricated MAPbI 3 nanowire photodetector under 660-nm illumination at the power density of 10.19 mW / cm 2 . (b) Rise/fall time indicated in one cycle. (c) Frequency response of the MAPbI 3 nanowire photodetector. (d) Stability test with the photodetector stored in air at a temperature of 20°C and humidity of 10%. The prepared photodetector exhibits stable response when the illumination is turned on and off.
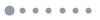
Fig. 5. Schematic illustration of the surface-initiated solution-growth strategy for preparing the single-crystalline MAPbI 3 nanowires. It includes mainly two steps. The first step is to coat a transparent lead acetate (PbAc 2 ) solid film based on the blade coating method, and the second step is to immerse the PbAc 2 film into the methyl ammonium iodide (MAI) solution at high concentration.
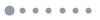
Fig. 6. (a)–(d) Optical images of MAPbI 3 nanowires grown from PbAc 2 thin film in relative humidities of 20%, 25%, 30%, and 35%. (e), (f) Corresponding absorption and PL spectra of MAPbI 3 nanowires grown in different humidities. (g) XRD pattern of as-grown MAPbI 3 nanowires with a comparison of these humidities.
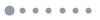
Fig. 7. Experimental XRD pattern of as-grown MAPbI 3 nanowires with a comparison of the simulated tetragonal phase MAPbI 3 . The as-grown MAPbI 3 nanowires exhibit approximately the same XRD pattern as the simulated one.
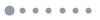
Fig. 8. (a) Experimental XRD pattern of the MAPbI 3 nanowires with a washing time of 20 s. (b) Simulated XRD pattern of MAI. Too short washing time (20 s) could cause a significant amount of MAI residue. The peaks at 19.8° and 29.7° in (a) indicate the existence of MAI in the final product. When the washing time is prolonged to 1 min, the pure single-crystalline MAPbI 3 product can be obtained.
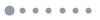
Fig. 9. Microscopic characterizations of the as-grown MAPbI 3 nanowires. (a) SEM image with an area of a single MAPbI 3 nanowire framed for the following EDS measurement. (b) EDS elemental mappings of C, Pb, N, and I, and the atomic ratios of different elements. The distribution of elements indicates that Pb and I elements are homogeneously distributed in the individual MAPbI 3 nanowire, and the atomic ratio between Pb and I elements is approximately 1∶3.
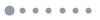
Fig. 10. AFM morphology of the PEDOT:PSS surface. It has an average RMS value of 0.16 nm, only a bit lower than that of the as-grown MAPbI 3 nanowires.
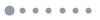
Fig. 11. (a) Schematic diagram of the fabricated MSM-type MAPbI 3 nanowire photodetector. (b) SEM image of a single MAPbI 3 nanowire sitting on the fingers of the interdigitated electrode. The planar metal–semiconductor–metal (MSM)-type photodetector with multiple nanowires adhered on top of the metal electrodes is fabricated. The effective photosensitive area is calculated by integrating all the areas of nanowires lying in between neighboring fingers of the electrode. At least 10 devices are fabricated for validating the calculation of the effective photosensitive area (∼ 90 μm 2 ).
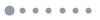
Fig. 12. Dark and photo I - V characteristic of a photodetector prepared by evaporating the electrodes on top of the as-grown MAPbI 3 wires.
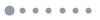
Fig. 13. Measured dark-current noise at various frequencies for the MAPbI 3 nanowire photodetector at 1 V bias. The calculated shot noise and thermal noise limits are also included for reference.
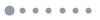
Fig. 14. (a), (b) Illumination spectrum with power densities of ∼ μW / cm 2 magnitude from the Xe lamp calibrated by the power meter and the corresponding spectral photocurrent response. The wavelength-dependent photodetection capability of MAPbI 3 nanowire photodetectors is studied using the Xe lamp as the light source for I - V characterizations. Illumination spectrum with power densities of ∼ μW / cm 2 magnitude from the Xe lamp calibrated by the power meter. It is found that our device exhibits a broadband photodetection ability from the wavelength of 300 nm to 800 nm. Here, the photocurrent at 500 nm with a power density of 29.8 μW / cm 2 is on the order of 1.15 nA, coincident with the LDR measurements shown in Fig. 2 (d).
|
Table 1. Device Performance Comparison of Different Perovskite Nanowire Photodetectors Measured at Room Temperaturea
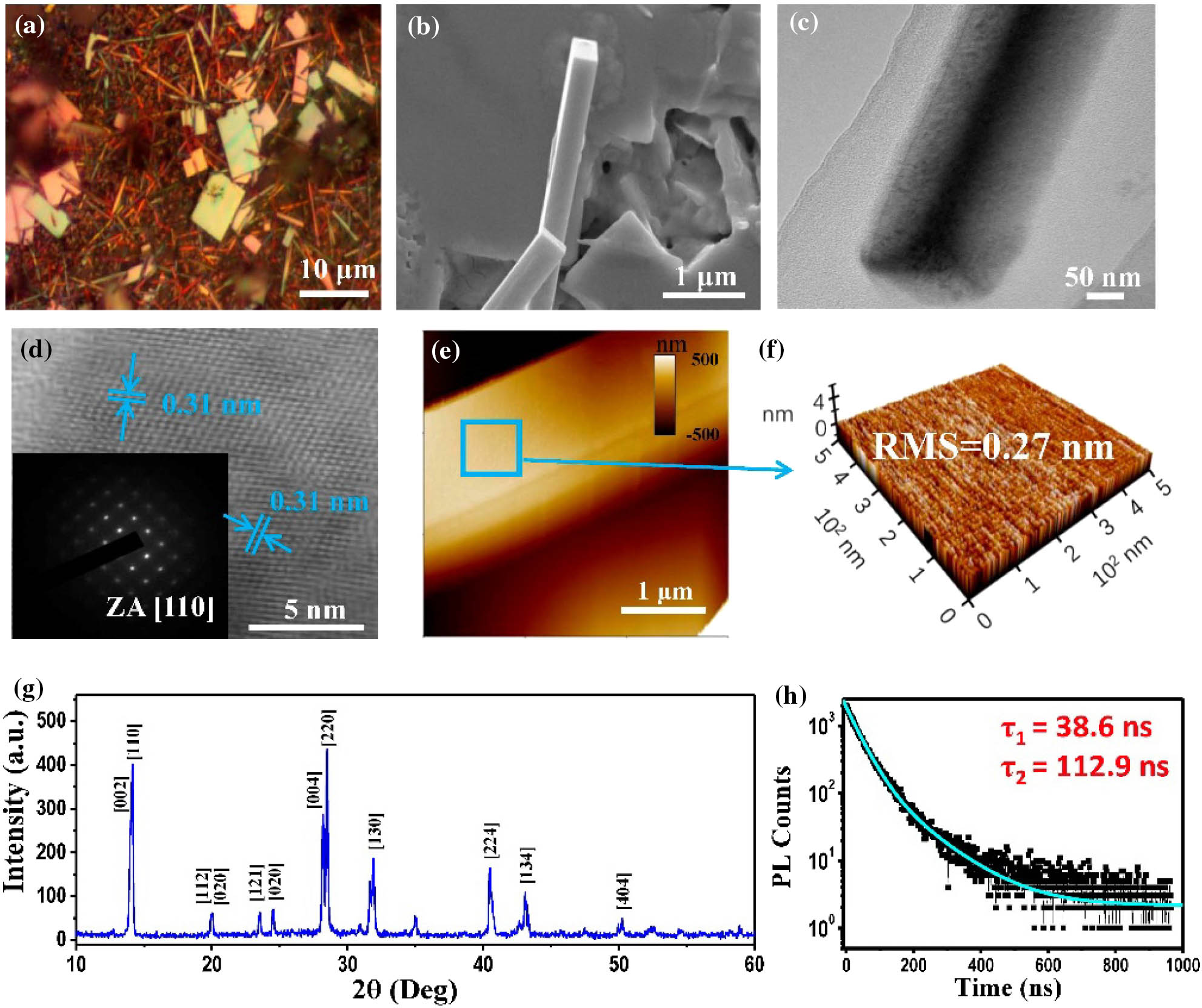
Set citation alerts for the article
Please enter your email address