Hui Ma, Haotian Yang, Bo Tang, Maoliang Wei, Junying Li, Jianghong Wu, Peng Zhang, Chunlei Sun, Lan Li, Hongtao Lin, "Passive devices at 2 µm wavelength on 200 mm CMOS-compatible silicon photonics platform [Invited]," Chin. Opt. Lett. 19, 071301 (2021)

Search by keywords or author
- Chinese Optics Letters
- Vol. 19, Issue 7, 071301 (2021)
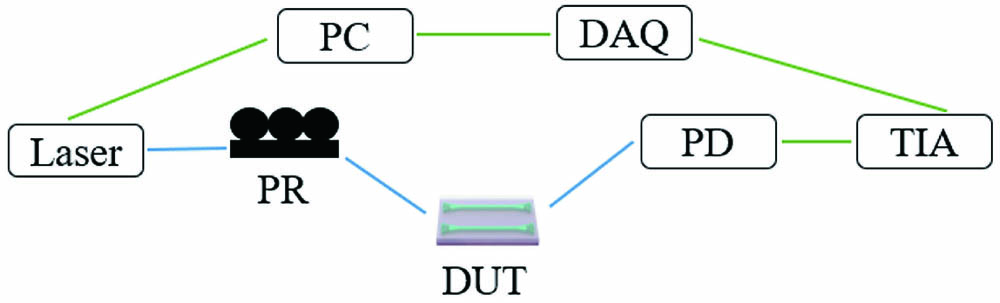
Fig. 1. Schematic diagram of the measurement system. PR, polarization rotator; DUT, device-under-test; PD, photodetector; TIA, trans-impedance amplifier; DAQ, data acquisition card; PC, personal computer.
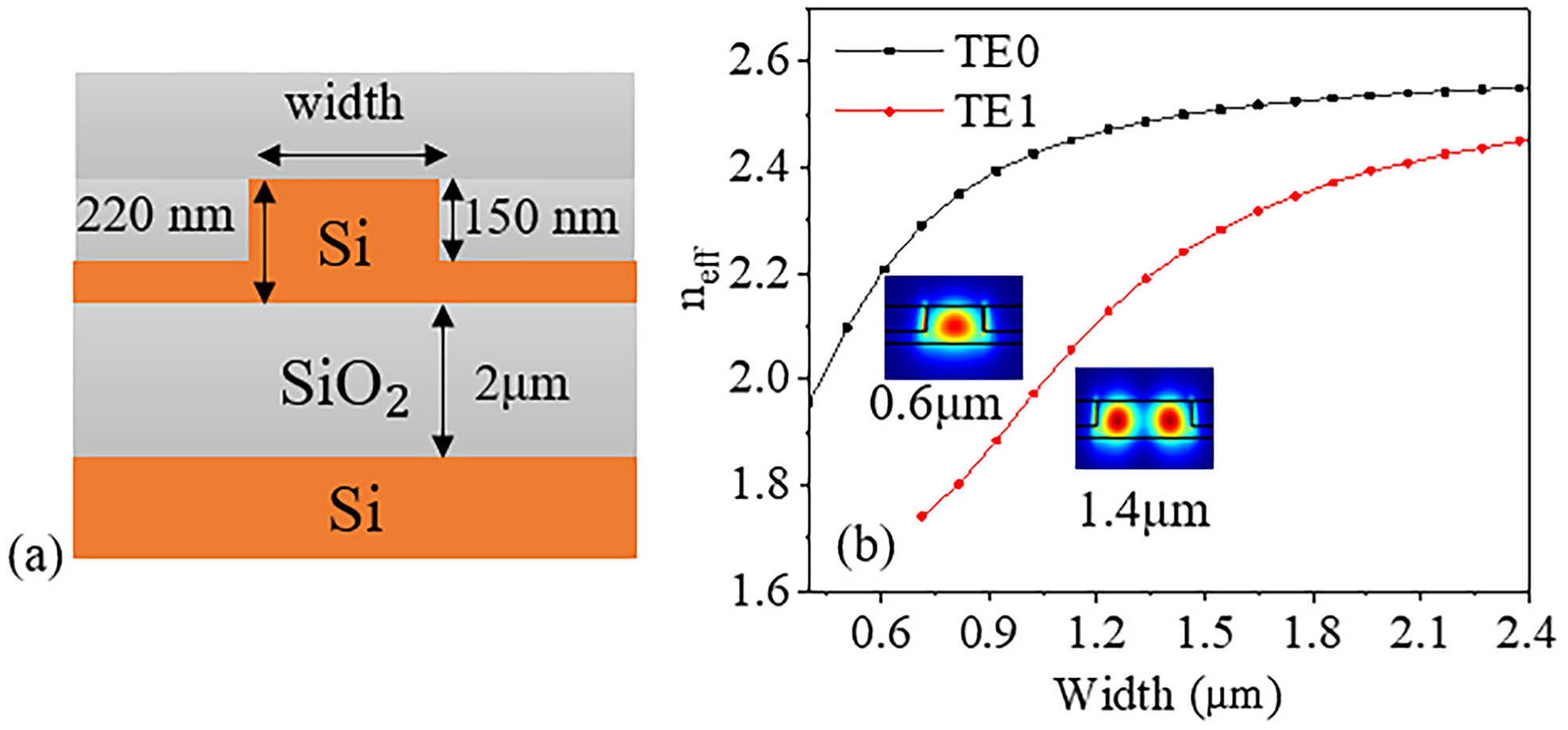
Fig. 2. (a) Cross-section diagram of the SOI ridge waveguide. (b) Calculated effective refractive index of the fundamental (black) and first-order (red) TE modes as a function of the ridge waveguide width at 2025 nm. Inset: the spatial distribution of the fundamental and second-order polarized optical modes into the SOI ridge waveguide with a width of 0.6 µm and 1.4 µm at 2025 nm, respectively.
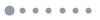
Fig. 3. (a) Measured spectral response of an MRR without doping. Inset: enlarged view of the measured resonance peak obtained by Lorentzian fitting and top-view SEM image of the MRR. (b) The measured spectral response of an MRR with light p-type doping.
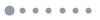
Fig. 4. (a) Simulated and measured coupling efficiency of the grating coupler. (b) Top-view SEM image of the fabricated GC.
![(a) Simulated transmission spectra of the crossing from port 1 to ports 2 and 3 [the port numbers are shown in (b-2)]. Inset: electric field distribution at 2025 nm. (b) Microscope and SEM images of the cascaded and single crossing. (b-1) Microscope view of cascaded crossing with numbers 15, 30, and 45, and the device structure for crosstalk test; (b-2) enlarged view of the structure for crosstalk test; (b-3) enlarged view of a single crossing. (c) Cut-back measurements for characterizing the insertion loss of crossings. (d) Measurements of the device crosstalk.](/Images/icon/loading.gif)
Fig. 5. (a) Simulated transmission spectra of the crossing from port 1 to ports 2 and 3 [the port numbers are shown in (b-2)]. Inset: electric field distribution at 2025 nm. (b) Microscope and SEM images of the cascaded and single crossing. (b-1) Microscope view of cascaded crossing with numbers 15, 30, and 45, and the device structure for crosstalk test; (b-2) enlarged view of the structure for crosstalk test; (b-3) enlarged view of a single crossing. (c) Cut-back measurements for characterizing the insertion loss of crossings. (d) Measurements of the device crosstalk.
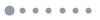
Fig. 6. (a) Simulated transmission spectrum of the 1 × 2 MMI at the wavelength of 2005–2035 nm. Inset: electric field distribution at the wavelength of 2020 nm. (b-1) Microscope view of cascaded 1 × 2 MMIs; the white numbers 0–7 represent the port number; (b-2) zoom-in SEM image of 1 × 2 MMI. (c) Measured transmission spectra of the 1 × 2 MMI at the wavelength of 2025 nm. (d) Total insertion losses as a function of the number of cascaded 1 × 2 MMIs at the wavelength range of 2020–2030 nm. The port numbers shown in (c) correspond to the number marked in (b-1), for example, the curve 0-1 shows the transmission spectrum from port 0 to 1.
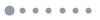
Fig. 7. (a) Optical image of the fabricated MZI. Ports 1 and 2 represent the input and output ports of the MZI. (b) Measured transmission spectrum of the MZI.
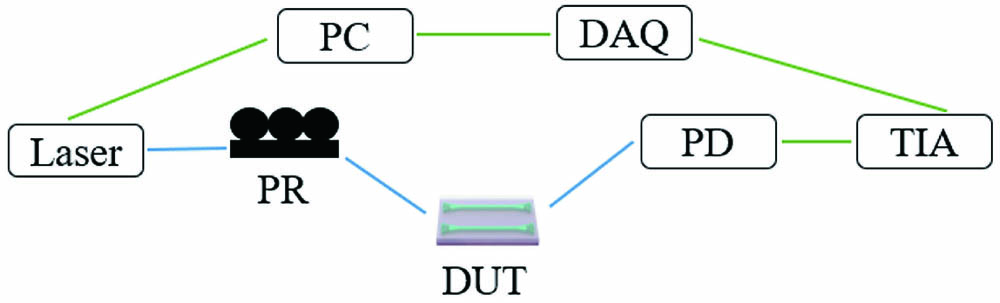
Set citation alerts for the article
Please enter your email address