Yu-Ran ZHEN, Jie DENG, Yong-Hao BU, Xu DAI, Yu YU, Meng-Die SHI, Ruo-Wen WANG, Tao YE, Gang CHEN, Jing ZHOU. Recent advances in on-chip infrared polarization detection[J]. Journal of Infrared and Millimeter Waves, 2024, 43(1): 52

Search by keywords or author
- Journal of Infrared and Millimeter Waves
- Vol. 43, Issue 1, 52 (2024)
![(a)Schematic diagram of tellurium(Te)crystal structure. Schematic diagram of the device structure. At room temperature,the incident power is 6.0 mW,and the net polarized photocurrent ΔIph is when the incident wavelength is 2.3 μm[29];(b)Schematic diagram of the unipolar barrier van der Waals heterostructure photodetector composed of b-AsP/WS2/b-AsP. Polar plots of linear polarization angle-dependent forward-bias-driven photocurrent and reverse-bias-driven photocurrent[30];(c)Schematic diagram of the full-Stokes polarization measurement setup. Comparison of helicity-dependent photocurrents at 0 V and -0.1 V for homostructure devices and monolayer MoS2[31];(d)Schematic of a twisted double bilayer graphene(TDBG)photodetector. Photovoltage(Vph)as a function of the quarter-wave plate(QWP)angle(θ)at different gate voltage biases(VBG,VTG)measured at T = 79 K and λ = 5 µm. Photovoltage function of VBG excited by 5 µm and 7.7 µm linearly polarized light(LP)when ψ = 165° and VTG = 5.2 V[32]](/richHtml/hwyhmb/2024/43/1/52/img_01.jpg)
Fig. 1. (a)Schematic diagram of tellurium(Te)crystal structure. Schematic diagram of the device structure. At room temperature,the incident power is 6.0 mW,and the net polarized photocurrent ΔIph is when the incident wavelength is 2.3 μm[29];(b)Schematic diagram of the unipolar barrier van der Waals heterostructure photodetector composed of b-AsP/WS2/b-AsP. Polar plots of linear polarization angle-dependent forward-bias-driven photocurrent and reverse-bias-driven photocurrent[30];(c)Schematic diagram of the full-Stokes polarization measurement setup. Comparison of helicity-dependent photocurrents at 0 V and -0.1 V for homostructure devices and monolayer MoS2[31];(d)Schematic of a twisted double bilayer graphene(TDBG)photodetector. Photovoltage(Vph)as a function of the quarter-wave plate(QWP)angle(θ)at different gate voltage biases(VBG,VTG)measured at T = 79 K and λ = 5 µm. Photovoltage function of VBG excited by 5 µm and 7.7 µm linearly polarized light(LP)when ψ = 165° and VTG = 5.2 V[32]
![(a)False-color scanning electron microscope image of a TaAs device. Along the a-axis and c-axis,the photocurrent varies with the angle of the quarter-wave plate[34];(b)Schematic experimental set-up for detecting the mid-infrared circular photogalvanic effect on a dual-gated monolayer WTe2 device. Polarization along the a-axis depends on the photocurrent[33];(c)The lattice structure of tellurium(Te). The quarter-wave plate-dependent photocurrent at the position of the maximum positive and negative response of the Te device under 10.6 μm(middle)and 4 μm(bottom)excitation[23];(d)Optical microscope image of the MoTe2 device. Crystal structure of Td-MoTe2. Anisotropic photocurrent response with a polarization extinction ratio of 2.72 for linearly polarized excitation at 10.6 μm[22]](/richHtml/hwyhmb/2024/43/1/52/img_02.jpg)
Fig. 2. (a)False-color scanning electron microscope image of a TaAs device. Along the a-axis and c-axis,the photocurrent varies with the angle of the quarter-wave plate[34];(b)Schematic experimental set-up for detecting the mid-infrared circular photogalvanic effect on a dual-gated monolayer WTe2 device. Polarization along the a-axis depends on the photocurrent[33];(c)The lattice structure of tellurium(Te). The quarter-wave plate-dependent photocurrent at the position of the maximum positive and negative response of the Te device under 10.6 μm(middle)and 4 μm(bottom)excitation[23];(d)Optical microscope image of the MoTe2 device. Crystal structure of Td-MoTe2. Anisotropic photocurrent response with a polarization extinction ratio of 2.72 for linearly polarized excitation at 10.6 μm[22]
![(a)Chiral hybrid perovskite(CHP)single-crystal array design for high-performance CPL direct photodetection[38];(b)Schematic of the photodetector. Crystal structure of(R- and S-α-PEA)PbI3. Circular dichroism(CD)and absorbance spectra of(R-,S-,and rac-α-PEA)PbI3 thin films[36];(c)Schematic diagram of a helical one-dimensional perovskite-based photodetector. J-V curves of(R-NEA)PbI3 device under LCP and RCP with a wavelength of 395 nm and an intensity of 1.0 mW cm-2[37];(d)Schematic diagram and crystal structure of vdW heterostructure photodetector device based on BP and chiral perovskite MPI. Electron and hole transfer process of MPI/BP heterostructure under 1550 nm illumination. Heterostructure output curves and individual BPs. Polar plot of the normalized polarization photocurrent measured at an illumination power of 100 μW and a wavelength of 1550 nm[39]](/Images/icon/loading.gif)
Fig. 3. (a)Chiral hybrid perovskite(CHP)single-crystal array design for high-performance CPL direct photodetection[38];(b)Schematic of the photodetector. Crystal structure of(R- and S-α-PEA)PbI3. Circular dichroism(CD)and absorbance spectra of(R-,S-,and rac-α-PEA)PbI3 thin films[36];(c)Schematic diagram of a helical one-dimensional perovskite-based photodetector. J-V curves of(R-NEA)PbI3 device under LCP and RCP with a wavelength of 395 nm and an intensity of 1.0 mW cm-2[37];(d)Schematic diagram and crystal structure of vdW heterostructure photodetector device based on BP and chiral perovskite MPI. Electron and hole transfer process of MPI/BP heterostructure under 1550 nm illumination. Heterostructure output curves and individual BPs. Polar plot of the normalized polarization photocurrent measured at an illumination power of 100 μW and a wavelength of 1550 nm[39]
![(a)SEM image of the cleaved facet of the cavity structure. SEM image of PCQWID,a grating plasmonic microcavity quantum well infrared detector. The relationship between the average intensity of the photocurrent measured at the wavelength of 14.2 ~ 14.9 µm and the polarization angle of the incident light[40];(b)Schematic of the chiral metamaterial and CPL detector. Experimentally measured circular dichroism spectra of the left-handed(LH,blue)and right-handed(RH,red)metamaterials[41];(c)HTN schematic and how it works. The ellipticity factor of the output beam of the helical traveling wave nanoantenna HTN and the experimental spectrum of the DOCP. Polarization state analysis at wavelengths of 1.55 μm and 1.64 μm[42];(d)Schematic illustration of the hybrid structure consisting of a chiral plasmonic metasurface and monolayer MoSe2. Optical absorption spectra of left-handed and right-handed plasmonic metasurfaces illuminated by light.[43]](/Images/icon/loading.gif)
Fig. 4. (a)SEM image of the cleaved facet of the cavity structure. SEM image of PCQWID,a grating plasmonic microcavity quantum well infrared detector. The relationship between the average intensity of the photocurrent measured at the wavelength of 14.2 ~ 14.9 µm and the polarization angle of the incident light[40];(b)Schematic of the chiral metamaterial and CPL detector. Experimentally measured circular dichroism spectra of the left-handed(LH,blue)and right-handed(RH,red)metamaterials[41];(c)HTN schematic and how it works. The ellipticity factor of the output beam of the helical traveling wave nanoantenna HTN and the experimental spectrum of the DOCP. Polarization state analysis at wavelengths of 1.55 μm and 1.64 μm[42];(d)Schematic illustration of the hybrid structure consisting of a chiral plasmonic metasurface and monolayer MoSe2. Optical absorption spectra of left-handed and right-handed plasmonic metasurfaces illuminated by light.[43]
![(a)Schematic diagram of 3D simulation of plasmonic microcavity quantum well infrared detector.(b)Resolution of Stokes parameters[44];(c)Schematic diagram of the asymmetric composite structure.(d)Absorption and reflection spectra of anisotropic dielectric composite structures under LCP and RCP illumination[45]](/Images/icon/loading.gif)
Fig. 5. (a)Schematic diagram of 3D simulation of plasmonic microcavity quantum well infrared detector.(b)Resolution of Stokes parameters[44];(c)Schematic diagram of the asymmetric composite structure.(d)Absorption and reflection spectra of anisotropic dielectric composite structures under LCP and RCP illumination[45]
![(a)Scanning electron microscope image of a thermopile element. Measured thermoelectric reactor emf voltage(black dots)as a function of incident light ellipticity angle χ compared to normalized S3 Stokes parameters at a wavelength of 7.9 µm and a light intensity of 270 W cm-2[53];(b)Device schematic for spin-controlled unidirectional plasmonic waveguide on-chip electrical detection. A Soleil-Babinet variable phase retarder was employed to convert linearly polarized laser radiation into left and right circular polarization states of intermediate elliptical and orthogonal linear polarization states[54];(c)Schematic of a metasurface-mediated graphene photodetector. I-V curves of the device under dark and light conditions[55];(d)Schematic of a nanoantenna-mediated semimetal photodetector. Measured(symbols)and fitted(dashed lines)photovoltage versus polarization angle for different gate voltages[56];(e)Symmetry analysis of the photoresponse of an achiral plasmonic nanostructure located on a graphene sheet. Measured I-V curve with drain-source bias. Illustration of a CPL-specific photodetector in a Poincaré sphere,where the photovoltage Vph depends only on the fourth Stokes parameter S3 of the incident light[57]](/Images/icon/loading.gif)
Fig. 6. (a)Scanning electron microscope image of a thermopile element. Measured thermoelectric reactor emf voltage(black dots)as a function of incident light ellipticity angle χ compared to normalized S3 Stokes parameters at a wavelength of 7.9 µm and a light intensity of 270 W cm-2[53];(b)Device schematic for spin-controlled unidirectional plasmonic waveguide on-chip electrical detection. A Soleil-Babinet variable phase retarder was employed to convert linearly polarized laser radiation into left and right circular polarization states of intermediate elliptical and orthogonal linear polarization states[54];(c)Schematic of a metasurface-mediated graphene photodetector. I-V curves of the device under dark and light conditions[55];(d)Schematic of a nanoantenna-mediated semimetal photodetector. Measured(symbols)and fitted(dashed lines)photovoltage versus polarization angle for different gate voltages[56];(e)Symmetry analysis of the photoresponse of an achiral plasmonic nanostructure located on a graphene sheet. Measured I-V curve with drain-source bias. Illustration of a CPL-specific photodetector in a Poincaré sphere,where the photovoltage Vph depends only on the fourth Stokes parameter S3 of the incident light[57]
![(a)SEM image of a polarimeter. Polar plot of photoresponse as a function of quarter-wave plate(QWP)rotation angle[58];(b)SEM image of the device. Simulations and experiments examine the intensity of the 0° and 135° linearly polarized light(LP)components as a function of the half-wave plate(HWP). Simulations and experiments examine the intensity of different QWP angles for left-handed circularly polarized light(LCP)and right-handed circularly polarized light(RCP)components[59];(c)False-color SEM image of a fiber end-face stack. One cycle photocurrent of the twisted BP cell as a function of QWP angle[60];(d)Schematic diagram of on-chip phase demodulation for high-speed coherent optical communication. The normalized output intensities of different waveguide ports for a single nanodisk element and two double nanodisk elements were experimentally measured and simulated[61];(e)Structural design of resonant thermoelectric photoresponse. Polarization-angle-dependent photoresponse simulations(lines)and measurements(symbols)for five typical devices. Simulated(line)and measured(symbol)photoresponses of four typical devices as a function of QWP angle[62]](/Images/icon/loading.gif)
Fig. 7. (a)SEM image of a polarimeter. Polar plot of photoresponse as a function of quarter-wave plate(QWP)rotation angle[58];(b)SEM image of the device. Simulations and experiments examine the intensity of the 0° and 135° linearly polarized light(LP)components as a function of the half-wave plate(HWP). Simulations and experiments examine the intensity of different QWP angles for left-handed circularly polarized light(LCP)and right-handed circularly polarized light(RCP)components[59];(c)False-color SEM image of a fiber end-face stack. One cycle photocurrent of the twisted BP cell as a function of QWP angle[60];(d)Schematic diagram of on-chip phase demodulation for high-speed coherent optical communication. The normalized output intensities of different waveguide ports for a single nanodisk element and two double nanodisk elements were experimentally measured and simulated[61];(e)Structural design of resonant thermoelectric photoresponse. Polarization-angle-dependent photoresponse simulations(lines)and measurements(symbols)for five typical devices. Simulated(line)and measured(symbol)photoresponses of four typical devices as a function of QWP angle[62]
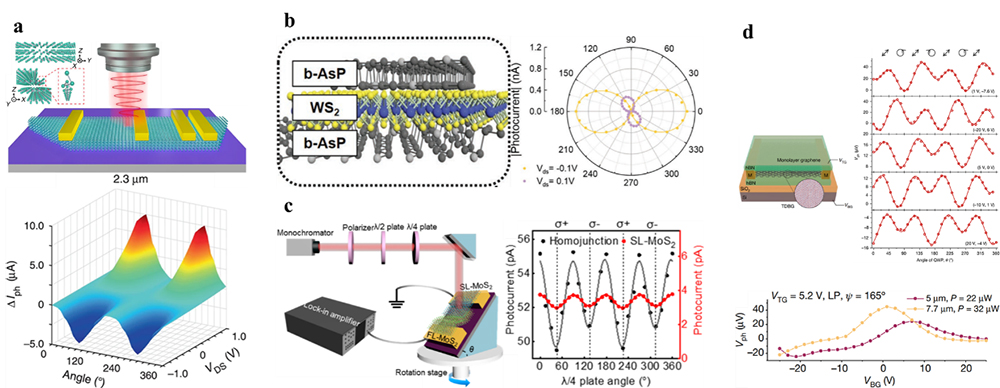
Set citation alerts for the article
Please enter your email address