Jiachen Li, Sigang Yang, Hongwei Chen, Xingjun Wang, Minghua Chen, Weiwen Zou, "Fully integrated hybrid microwave photonic receiver," Photonics Res. 10, 1472 (2022)

Search by keywords or author
- Photonics Research
- Vol. 10, Issue 6, 1472 (2022)
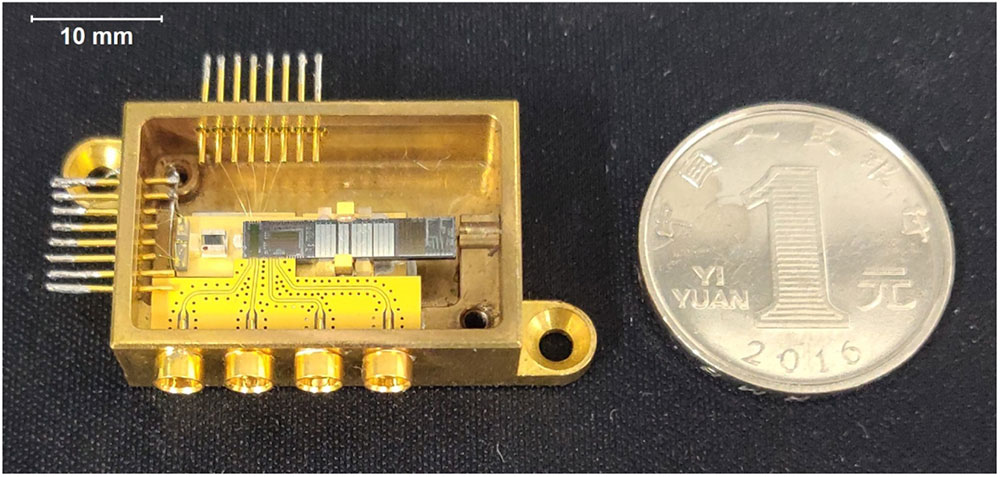
Fig. 1. Packaged FIH-MWPR module with a compact volume of 30 mm × 20 mm × 10 mm .
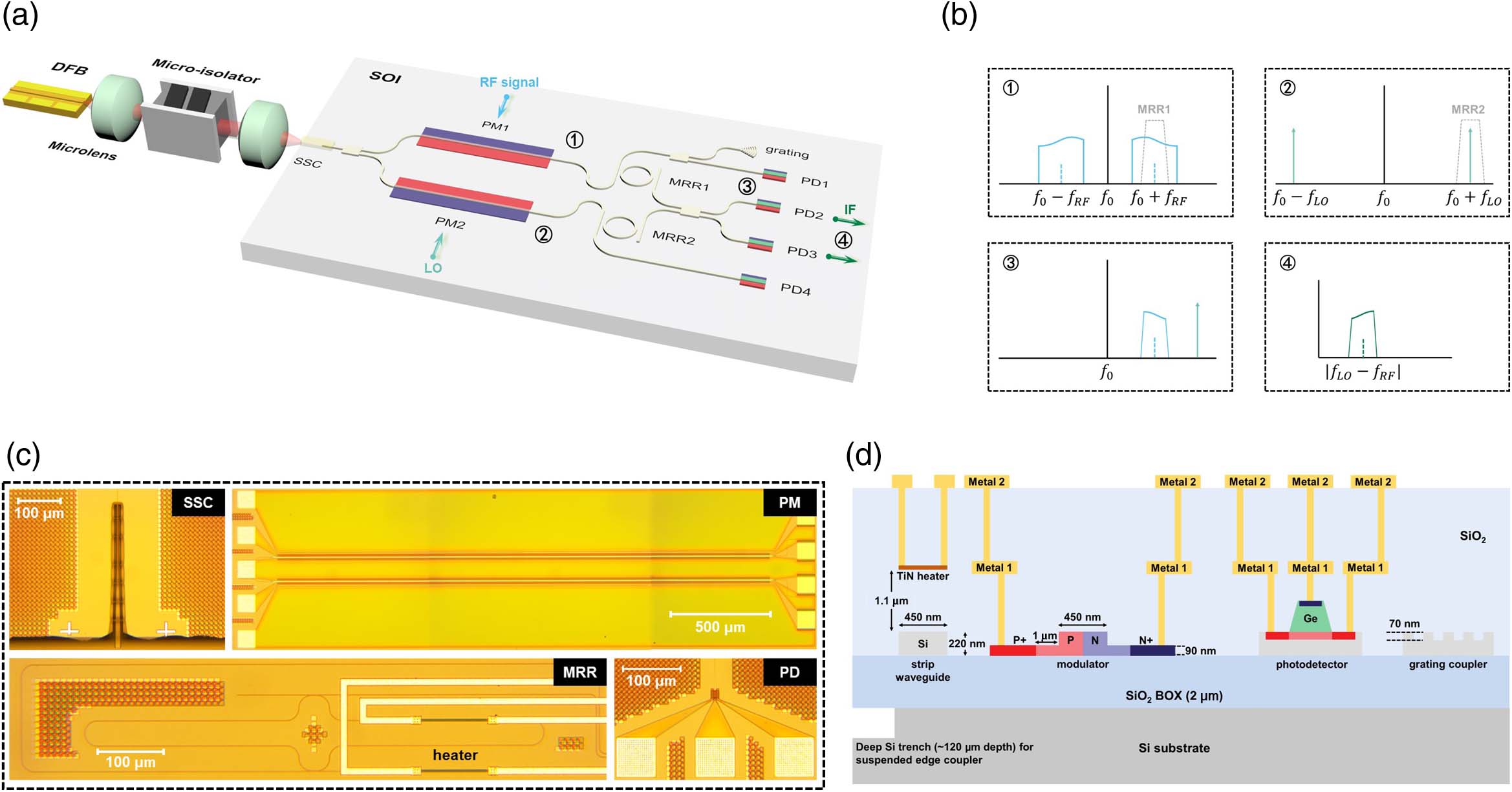
Fig. 2. (a) Schematic diagram of the proposed FIH-MWPR. DFB, distributed feedback semiconductor laser; SSC, spot-size converter; PM, phase modulator; MRR, microring resonator; PD, photodetector. (b) Spectra at several key points labeled in the system. 1, 2, and 3 are optical spectra, and 4 is the electrical spectrum. The signal–signal beat interference (SSBI) at 4 is ignored. (c) Optical microscope images of key elements on the fabricated SOI chip. (d) Cross-sectional schematic view (not to scale) of the SOI platform.
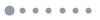
Fig. 3. Normalized measured E/O modulation responses of (a) the unpackaged on-chip phase modulator and (b) the packaged phase modulator under various bias voltages.
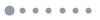
Fig. 4. (a) Measured MRR notch response (green, dots), simulated MRR bandpass response (blue, solid line), and the Lorentzian fitting (green, dashed line) of the measured notch response. (b) The MRR resonance wavelength is tuned with the increasing heating power. (c) Temporal waveform of the square-wave electrical driving signal applied on the TiN microheater. (d) Measured temporal optical response with a fall/rise time of ∼ 66 and ∼ 15 μs .
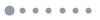
Fig. 5. (a) Measured responsivities of on-chip photodetectors under various bias voltages. (b) Normalized measured O/E responses of on-chip photodetectors under various bias voltages.
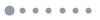
Fig. 6. (a) Measured optical spectrum of the employed InP-based DFB semiconductor laser. (b) Frequency noise measurement of the DFB laser showing a Lorentzian linewidth of ∼ 320 kHz .
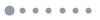
Fig. 7. (a) Measured electrical spectrum (RBW: 100 kHz, VBW: 1 kHz) of the down-converted IF signal, with the two-tone RF signal centered at 14 GHz (14.001 and 13.999 GHz) and the LO (20 dBm) centered at 14.2 GHz. (b) Measured output power of the IF fundamental tones and the IMD3 tones under different RF input power. (c) Measured link gain of the FIH-MWPR over the frequency range from 2 to 18 GHz. (d) Measured noise floor of the FIH-MWPR over the frequency range from 2 to 18 GHz. (e) Measured NF of the FIH-MWPR over the frequency range from 2 to 18 GHz. (f) Measured SFDR of the FIH-MWPR over the frequency range from 2 to 18 GHz.
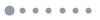
Fig. 8. Link gain, NF, and SFDR measured as functions of IF. Here, the RF input signal is fixed at 14 GHz, while the LO (20 dBm) is tuned from 14.1 to 17 GHz.
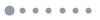
Fig. 9. (a) Experimental setup for evaluating the system performance of the FIH-MWPR as a de-chirp receiver. The inset shows the de-chirping principle. MZM, Mach–Zehnder modulator; EDFA, erbium-doped fiber amplifier; OBPF, optical bandpass filter; OC, optical coupler; VOA, variable optical attenuator; PD, photodetector; AWG, arbitrary waveform generator; LNA, low noise amplifier; EBPF, electrical bandpass filter; ADC, analog to digital converter; DSP, digital signal processor. (b) Instantaneous time-frequency diagrams of generated LFM radar echo signals from the fiber-delay-based radar target emulator at different frequency bands (S-, C-, X-, and Ku-bands). (c) The normalized electrical spectra of de-chirped IF signals generated by the FIH-MWPR, with the LFM radar signals located at different frequency bands (S-, C-, X-, and Ku-bands). The insets show the zoom-in views around the main peaks. (d) Measured frequencies of the de-chirped IF signals under different fiber delay distances, with the LFM radar signals located at different frequency bands (S-, C-, X-, and Ku-bands).
|
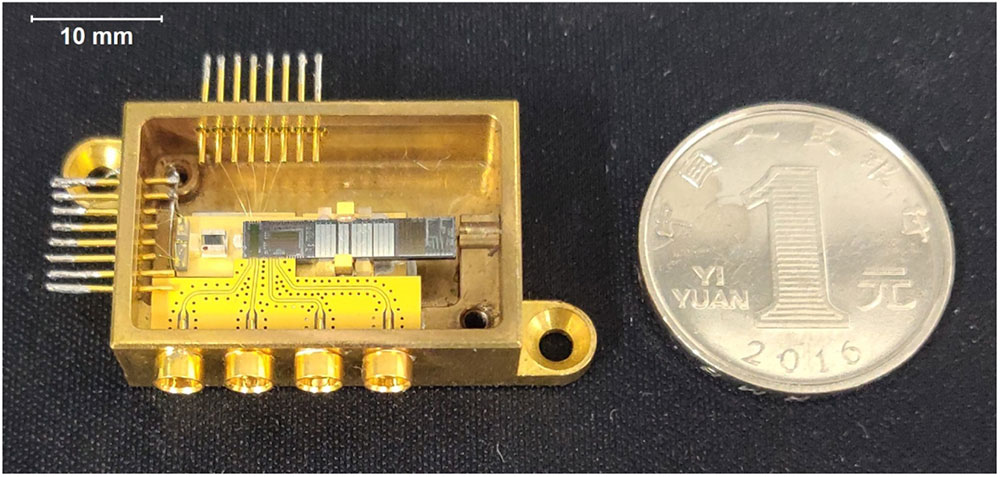
Set citation alerts for the article
Please enter your email address