Kapil Debnath, David J. Thomson, Weiwei Zhang, Ali Z. Khokhar, Callum Littlejohns, James Byers, Lorenzo Mastronardi, Muhammad K. Husain, Kouta Ibukuro, Frederic Y. Gardes, Graham T. Reed, Shinichi Saito, "All-silicon carrier accumulation modulator based on a lateral metal-oxide-semiconductor capacitor," Photonics Res. 6, 373 (2018)

Search by keywords or author
- Photonics Research
- Vol. 6, Issue 5, 373 (2018)
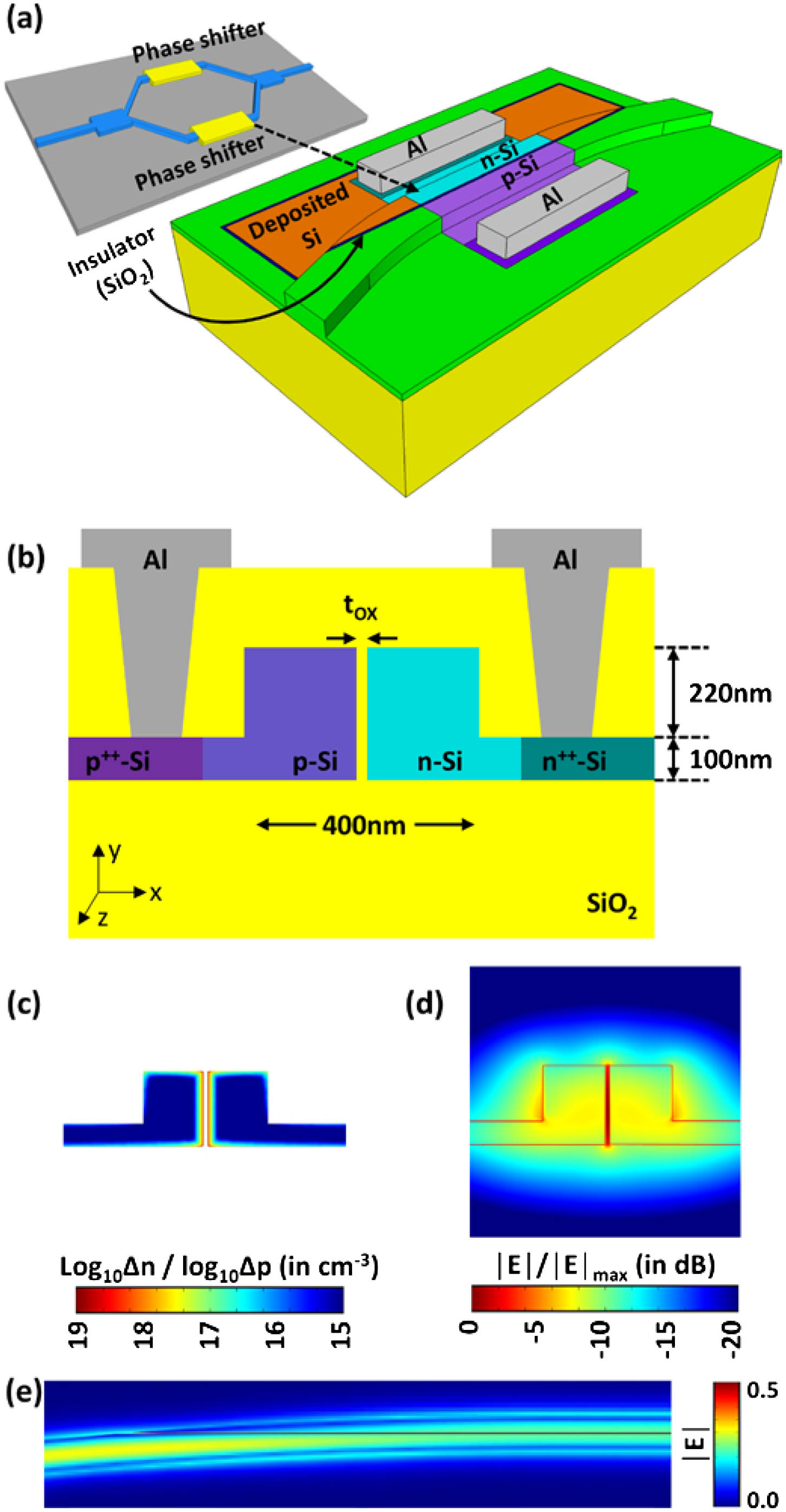
Fig. 1. (a) Schematic of the Si / SiO 2 / Si MOS-capacitor phase shifter. The relative position of the phase shift in an MZI interferometer is highlighted in yellow. (b) Cross-sectional view of the MOS-capacitor structure used for designing the phase modulator. The width and height of the rib waveguide are 400 nm and 320 nm, respectively, and the slab height is 100 nm. The n-Si and p-Si regions have a doping concentration of 1 × 10 18 cm − 3 . The n++-Si and p++-Si regions have a doping concentration of 1 × 10 20 cm − 3 and are positioned 900 nm away from the center of the waveguide. The oxide thickness t ox is varied in the design to optimize device performance. (c) Excess carrier concentration distribution (in log scale) due to an applied bias of 4 V. (d) Normalized dominant electric field (| E x | ) profile (in dB) of the fundamental TE mode centered around the oxide slot region. (e) Simulated electric field (| E x | ) propagation from the rib waveguide region to the slot-waveguide region.
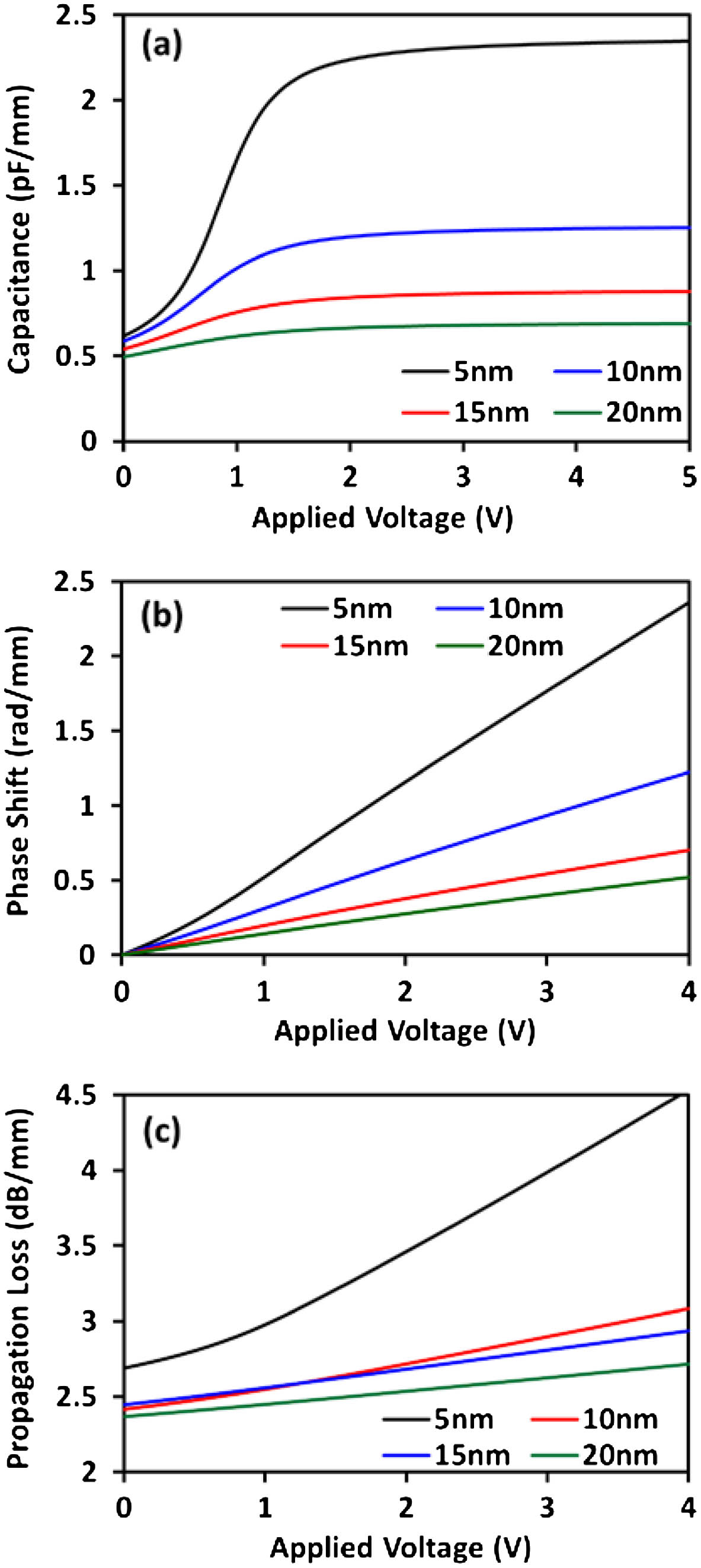
Fig. 2. (a) Calculated change in device capacitance (in pF/mm) as a function of applied voltage. Since the modulator operates in accumulation regime, only positive bias is shown. The capacitance increases with reducing oxide thickness (t ox ). (b) Calculated phase shift (in rad/mm) as a function of applied voltage. The phase shift per unit length increases with reducing oxide thickness and applied voltage. (c) Calculated propagation loss (in dB/mm) as a function of applied voltage. The loss increases with reducing oxide thickness and applied voltage.
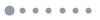
Fig. 3. (a) Cross-sectional SEM image of the fabricated modulator across the active region. (b) Magnified view of the MOS capacitor at the rib section, where the oxide layer is clearly visible. (c) Optical image of the fabricated MZI modulator with 500-μm-long phase shifter.
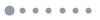
Fig. 4. (a) Measured transmission spectra of the MZI modulator with 500-μm-long phase shifter as a function of applied voltage. The signal probe was connected to n-Si electrode while the ground probe was connected to the p-Si electrode. The output power of the modulator was normalized to a reference Si waveguide with same length. (b) Measured phase modulation (in radian) and extracted modulation efficiency V π L π (in V · c m ) as a function of applied voltage. (c) Measured eye diagrams at 20 Gbit / s and 25 Gbit / s . In all cases the driving voltage was 6 V with a DC bias of 3 V.
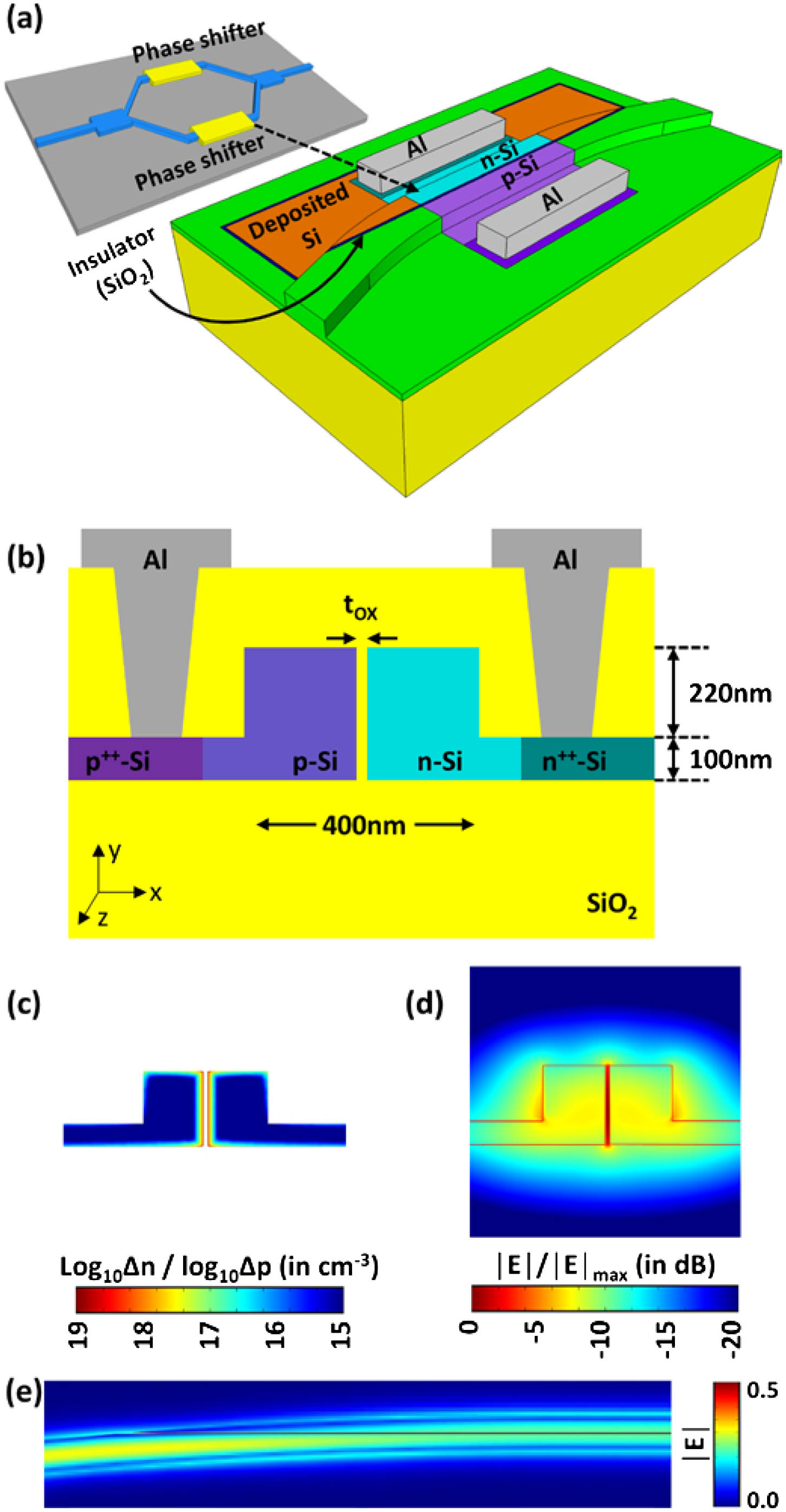
Set citation alerts for the article
Please enter your email address