
- Photonics Research
- Vol. 10, Issue 7, 1543 (2022)
Abstract
1. INTRODUCTION
Varifocal imaging is an essential function in many optics applications, such as biomedicine, photography, smartphones, and virtual reality [1–3]. Most demonstrated optical devices to date adopt mechanical axial movement of several lenses over specific distances along the optical axis to realize the varifocal function, which has many problems such as having a large volume, being expensive, showing mechanical wear, and being slow [4,5]. These devices left much to be the miniaturization modern optical imaging desired. To meet the demand for miniaturization, considerable efforts have been made to develop compact varifocal lenses. Adaptive lenses provide a more compact, faster response speed, and lighter-weight alternative than traditional varifocal lens assemblies [6,7]. According to the difference in operation mechanisms, adaptive lenses can be broadly classified into two categories: liquid lenses and liquid crystal lenses. The liquid lenses change the focal length by changing the curvature radius of the refractive surface [8]. Although many miniaturized liquid lenses have been proposed, including the membrane-less [9–11] and membrane-structured liquid lenses [12,13], these lenses suffer from some problems due to the intrinsic characteristics of the liquid, such as being sensitive to temperature and gravity, leaking easily, and having an unstable optical axis [14–17]. Liquid crystal lenses are subject to a graded refractive index distribution by changing the orientations of the directors under the application of an inhomogeneous electric field, thereby controlling the focal length [18,19]. However, there are some inherent limitations of the liquid crystal lenses, such as polarization dependence, small aperture, and unavoidable electrode inclusion on the optical path [20]. Besides liquid lenses and liquid crystal lenses, some other means can also be used to realize varifocal functionality [21–23].
Alvarez lenses, independently developed by Alvarez [24] and Lohmann [25] a few decades ago, are different types of lenses with varifocal capability. They are composed of a pair of lens elements, which have complementary cubic surface profiles. The Alvarez lenses provide a wide optical power tuning range through small lateral displacements perpendicular to the optical axis, rather than the mechanical movement along the optical axis that is employed in the traditional varifocal lenses. Thus, the Alvarez lenses have the potential to achieve a more compact structure, a wider varifocal range, a faster response time, more ease of packaging, and higher stability, which can be a powerful alternative for miniature imaging systems [26]. Moreover, due to no liquid involved, the Alvarez lenses show several remarkable advantages in terms of temperature sensitivity, response speed, and environmental stability. When the two lens elements of the Alvarez lenses are aligned in registration, the resulting phase profile is null, and the Alvarez lenses do not focus light, which can be interpreted as having an infinite focal length. When the lens elements have a lateral movement, one with respect to the other, perpendicular to the optical axis, it allows the Alvarez lenses to change from a negative power lens to a positive power lens depending on the lateral movement direction [27–29].
Although the Alvarez lenses concept has been proposed for many years, it has not been widely used for a long time mainly due to fabrication challenges of the required cubic freeform surface [30]. Fortunately, benefiting from the advanced optical manufacturing technology, many optical systems using Alvarez lenses have also been studied [31,32]. The emerging diamond micromachining technology enables the manufacturing of the required surfaces of the Alvarez lenses with adequate depth modulation and surface finish. However, actuating the Alvarez lens with a large lateral movement in a simple method is a concern for the varifocal lenses based on the Alvarez lenses. The focal length variability of the Alvarez lenses has been realized by MEMS-driven technology [33]. The lateral displacement range of the micro-electromechanical systems (MEMS)-driven technology is usually less than 500 μm. A wide range of focal length tuning is difficult to achieve due to the small lateral displacement. Although some amplifiers can be used to enlarge lateral displacement, the size of the amplifier equipment is relatively large. A method of manually actuating the lateral movement of the Alvarez lenses is proposed [34,35], but the tuning speed and precision of the focal length cannot be scaled to the modern varifocal applications. Therefore, it is necessary to propose a novel driving mechanism of the Alvarez lenses in order to overcome the disadvantages of traditional actuation methods, including small displacement, slow speed, and complex structure. Moreover, when the interest region does not lie in the center of the field of view, it is necessary to align the central field of view with the region of interest because of the observation habit of the human eye and the high imaging quality in the central field of view for imaging systems [36]. The traditional method is to move the imaging target or to manually adjust the lens to make the region of interest located in the center of the field of view, which has the problems of low accuracy, slow response speed, and low efficiency [37].
Sign up for Photonics Research TOC. Get the latest issue of Photonics Research delivered right to you!Sign up now
To actuate the Alvarez lenses with a large displacement and high speed in a simple method, we present novel lens elements based on the Alvarez lenses actuated by dielectric elastomer (DE). The lens elements are composed of the varifocal component and the scanning component. The lens elements have a variable focus function based on the Alvarez lens structure and DE actuator and a scanning function based on the DE-based four-quadrant actuators. The proposed lens elements are used to develop an ultra-wide varifocal imaging system with selectable region of interest capability [38]. In particular, we show that the imaging system with such properties can be obtained by combining an emerging class of “artificial muscle” materials with the varifocal technology of Alvarez lenses.
2. DESIGN AND FABRICATION
The proposed lens elements comprise a varifocal component and a scanning component. The varifocal component is placed and fixed at the center of the scanning component, as shown in Fig. 1(a). The image of the fabricated lens elements is shown in Fig. 1(b). The varifocal component and scanning component are both driven by DE actuators. Within the family of electroactive polymers, DEs are quickly emerging as a top choice of “smart” materials for new kinds of soft actuators capable of high strain, high energy density, high efficiency, fast response speed, noise-free operation, high resilience, and lightweight [39]. When the driving voltage is applied to the DE membrane, the thickness of the DE decreases, and the surface increases under Maxwell stress [40].
Figure 1.(a) Architecture of the proposed lens elements. The proposed lens elements are mainly composed of a varifocal component and a scanning component. The two components are both driven by the DE actuators. The varifocal component is mainly composed of four acrylic frames, two lens elements of the Alvarez lenses, and two DE membranes. The lateral relative movements of the two lens elements can be realized by applying driving voltages to the right and left compliant electrodes on the DE membrane. Hence, the focal length of the varifocal component can be altered. The DE membrane of the scanning component is divided into four quadrants. The scanning component allows the varifocal component to move in different directions and makes the varifocal component images the region of interest in the center of the field of view by applying voltages on the DE-based four-quadrant actuators. (b) The photograph of the fabricated proposed lens elements with a selectable region of interest capacity using Alvarez lenses actuated by the DE membrane. The magnified picture is the fabricated Alvarez lenses viewed along the optical axis. (c) In the rest state (driving voltage is off), the two lens elements of the Alvarez lenses do not have lateral displacement, and the Alvarez lenses are equivalent to flat plates, i.e., parallel light enters and parallel light exits. (d) In the activation state (driving voltage is on), the focal length of the varifocal component is changed because the two lens elements move relative to each other when an actuation voltage is applied to the DE membrane. The exit light is focused by the varifocal component. (e) The three-dimensional view of the free-form surface of one lens element of the Alvarez lenses.
The proposed varifocal component is mainly constructed from four acrylic frames, two lens elements of the Alvarez lenses, and two DE membranes. The two DE membranes are coated with compliant electrodes on local areas along both the left and right sides of the Alvarez lenses. The Alvarez lenses consist of two lens elements, where each lens element has a plane-freeform structure, i.e., one plane surface side and one free-form surface side described by a cubic polynomial equation, which can be given by [24,25,41]
Initially, the two lens elements are precisely aligned along the optic axis and the cubic surfaces are inverted concerning each other. When there is no driving voltage applied to the compliant electrodes, the two lens elements act as a plate of constant thickness and thus have an infinite focal length (ignoring the air gap), as depicted in Fig. 1(c). When the driving voltage applied to the compliant electrodes is on, the electrostatic attraction of the DE membrane produces radially compressive force directing towards the lens at the center and subsequently pulls the lens elements to move relatively. The relative lateral movements of the two lens elements make the focal length of the varifocal component change, as shown in Fig. 1(d). The varifocal component acts as a convex lens when the first lens element moves in the negative
The proposed scanning component is mainly composed of two acrylic frames and one DE membrane. The DE is sandwiched by the two frames. The DE membrane is divided into four quadrants and both sides of the four quadrants are coated with compliant electrodes. These four quadrants are isolated by enough gaps to prevent electrical connection. When the driving voltage is applied to the compliant electrodes of a quadrant, the DE membrane of the actuated quadrant expands in the lateral direction because it is an incompressible material, i.e., its volume does not change. Hence, the varifocal component moves in the lateral direction. By applying actuation voltages to different quadrants, the scanning component allows the varifocal component to move in different directions and makes the varifocal component images the region of interest in the center of the field of view. Therefore, the proposed lens elements can both focus and image the region of interest by applying voltages to the compliant electrodes of the varifocal component and scanning component, respectively.
The fabrication processes of the proposed lens elements are described as follows. First, two lens elements of the Alvarez lenses with a diameter of 6.0 mm are fabricated by a single-point diamond turning technique (Nanoform 250, Precitech) with a programming resolution of 0.01 nm, as shown in the magnified picture in Fig. 1(b). The two lens elements are fabricated from acrylic material with a refractive index of 1.47. The three parameters in Eq. (1), describing the freeform of the two lens elements of the Alvarez lenses are
Second, the proposed varifocal component can be divided into upper and lower parts. The explosive view of the lower part is shown in Fig. 2(a). Two 2-mm-thick polymethyl methacrylate (PMMA) frames with an inner diameter of 20 mm and an outer diameter of 24 mm are fabricated by a laser engraving machine (4060, Ketailaser Company). The DE membrane (VHB4905, 3M Company) is sandwiched by the two acrylic frames, and the top and bottom sides of the local areas of the DE membrane along the
Figure 2.Schematic illustration of the fabrication procedure of the proposed lens elements. (a) The main components of the lower part of the varifocal component. The DE membrane (VHB4905, 3M Company) is pre-stretched with a ratio of 200%. The top and bottom sides of two local areas of the VHB4905 along the
Third, the composition of the scanning component is shown in Fig. 2(d). It is mainly composed of two annular acrylic frames (top frame and bottom frame) and one DE membrane with four quadrants (
3. EXPERIMENTS AND RESULTS
According to Eq. (2), when the two parameters (
Figure 3.(a) Relationship between the lateral displacement of the lens element of the Alvarez lenses and the driving voltage applied to the active compliant electrode. (b) The experimental and theoretical focal lengths of the varifocal component. The experimental focal length is measured by the magnification method, and the theoretical focal length is evaluated by the lateral displacement according to Eq. (
The range of focal length is an important parameter for evaluating the varifocal lens. To qualitatively assess the focal length of the varifocal component based on the Alvarez lenses, the range of the focal length is measured by using the magnification method. A young embryo of a
Compared with the traditional varifocal lens, which achieves focusing tuning ability by moving the lens along the optical axis using mechanical parts, the proposed varifocal component tunes the focal length through the lateral displacement of the two lens elements of the Alvarez lenses. Without the movement of any mechanical parts, the proposed varifocal component can focus the objects and obtain clear images at different distances. To qualitatively assess the tunable focal length performance of the proposed varifocal component based on the Alvarez lenses actuated by the DE membrane, images of three objects located at different distances from the varifocal component are obtained by the microscope. The proposed lens elements are mounted at a fixed distance of 3.0 mm from the microscope, and the driving voltage is adjusted until the sharpest image of each object is obtained. The experimental setup and captured images are shown in Fig. 4. Three 1-mm-thick vitreous tissue sections, including the daphnia section, the fibrous connective section, and the young embryo of a
Figure 4.(a) Demonstration of adaptive focusing by viewing three tissue sections placed at different distances from the proposed lens elements. Three tissue sections, including the daphnia (Tissue section 1), the fibrous connective (Tissue section 2), and the young embryo of
The tuning speed of the focal length is an important parameter for evaluating the dynamic performance of the proposed lens elements. Fast-changing focal length ability is significant for many application scenarios, such as biological cell tracking, smartphones, and aerospace [42,43]. We test the response time of the dynamic performance of the proposed varifocal component. The experimental schematic is shown in Fig. 4(b). A laser light beam is generated by a laser (MGL-III-532, Changchun New Industries Optoelectronics Technology Company) and collimated by a beam expander (GCO02501, Daheng Optics). The collimated beam passes through a diaphragm with a 5-μm-pinhole (GCT-060201, Daheng Optics) and focuses on the photodetector (PDA36A-EC, Thorlabs) through the proposed varifocal component. The photodetector is used to record the light intensity of the laser beam passing through the pinhole. The focal length can be tuned when a driving voltage is applied to the compliant electrode of the DE membrane, which makes the recorded light intensity change and then the recorded voltage different. The dynamic response, when the input voltage generated from the function generator (DG1062, RIGOL Technologies) is a square signal with a frequency of 1 Hz, an amplitude of 3.0 V, and a duty cycle of 50%, is shown in Fig. 4(c). The input voltage is amplified by a power amplifier (PA1011, RIGOL Technologies) and then is applied to the compliant electrode of the DE membrane. The response time of the proposed adaptive lens is obtained from the local magnified area in Fig. 4(c). The rise and fall times are regarded as the time consumption from the initially recorded voltage to 90% of the maximum recorded voltage and from the maximum recorded voltage to 90% of the initially recorded voltage [44], respectively, as shown in Fig. 4(d). We can observe that rise and fall times of the proposed ultra-varifocal component are 160 ms and 295 ms, respectively. Because the DE used for the scanning component has the same stretch ratio as the varifocal component, the response time of the scanning component is in the same order of magnitude as the varifocal component. There are several feasible ways to increase the response speed, including reducing the mass of the two lens elements of the Alvarez lens and using the DE membrane with high stiffness and a high dielectric permittivity.
When the interesting region is not in the center of the field of view of the imaging system, it is necessary to align the central field of view with the region of interest because of the observation habit of the human eye and the high imaging quality in the central field of view. To verify the selectable region of interest capacity, an experimental imaging system based on the proposed lens elements is built to realize ultra-wide varifocal imaging with a selectable region of interest, as shown in Fig. 5(a). A biological intestine tissue section is located at fixed distances of 3.0 mm from the proposed varifocal lens element and is imaged on the microscope through the proposed varifocal lens element. By applying driving voltages to four-quadrant actuators, the scanning component allows the varifocal component to move in different directions and endows the varifocal component with the capacity of making the selectable region of interest in the center of the field of view. At the initial state, there is no driving voltage applied to any quadrants of the scanning component and the varifocal component, and the image is shown in Fig. 5(c). To scan the imaging area and change the focal length, a driving voltage of 4.0 kV generated from the voltage-stabilized source 2 is applied to the varifocal component, and a driving voltage of 5.0 kV generated from the voltage-stabilized source 1 is applied to different quadrants (
Figure 5.(a) Experimental schematic for evaluating the selectable region of interest capacity of the ultra-wide varifocal imaging system based on the proposed lens elements. By applying actuation voltage on the varifocal component using voltage-stabilized source 2 while applying actuation voltage on the scanning component using voltage-stabilized source 1, the varifocal component can magnify the object, and the scanning component allows the varifocal component to move in different directions, which endows the varifocal component with the capacity of making the selectable region of interest in the center of the field of view. (b) The experimental schematic for characterizing the image resolution of the ultra-wide varifocal imaging system. (c) The image at the rest state. There is no driving voltage applied to any quadrants of the scanning component and the varifocal component. (d)–(g) Images at different actuation states when different driving voltages are applied to the four-quadrant actuators of the scanning component. (h) The image of the resolution target at the rest state. There is no driving voltage applied to the varifocal component. (i)–(l) The images of the resolution target at different actuation states.
To characterize the image resolution of the ultra-wide varifocal imaging system with a selectable region of interest, a USAF negative resolution target (USAF1951, Edmund Optics) is located at a fixed distance of 3.0 mm from the imaging system, and the image is captured by the microscope through the proposed lens elements. The experimental setup is shown in Fig. 5(b). At the initial state, when there is no driving voltage applied to the varifocal component, the image is shown in Fig. 5(h). Figure 5(h) shows that the proposed lens elements could resolve group 7 and element 1, and the corresponding imaging resolution is approximately 128 lp/mm. When driving voltages of 4.0 kV and 3.0 kV are applied to actuate the first lens element of the Alvarez lenses when it moves along the positive
4. CONCLUSIONS
In summary, we develop lens elements with a wide focal length tuning range and selectable region of interest capacity. The focal length variation of the proposed lens elements is up to 30.5 times, where the maximum focal length is 181 mm and the minimum focal length is 5.94 mm. The proposed lens elements are composed of the varifocal component and the scanning component. The actuation of the two components is realized by the DE membrane. The function of the variable focal length is achieved based on the Alvarez lenses. The large deformation generated by the DE actuators permits lateral displacement of the Alvarez lenses up to 1.145 mm. The proposed lens elements can both change the focal length and select the region of interest without mechanical movement. The response time of the varifocal component is tested, and the results show that rise and fall times are 160 ms and 295 ms, respectively. Based on the proposed lens elements, an imaging system is built to realize ultra-wide varifocal imaging with a selectable region of interest. The imaging resolution of the system is approximately 181 lp/mm. The demonstrated lens elements provide a pathway to develop ultra-wide varifocal optical imaging systems while retaining high-resolution and fast response speed by combining lateral displacement characteristics of Alvarez lenses and the large deformation ability of the DE membrane. The proposed lens elements still have some drawbacks, such as high voltage actuation, large aberration, and a narrow field-of-view. Further research, including reduction of the actuation voltage and aberrations, enhancing the field-of-view, and implementing of potential imaging applications, will be conducted.
References
[1] L. Dong, A. K. Agarwal, D. J. Beebe, H. Jiang. Adaptive liquid microlenses activated by stimuli-responsive hydrogels. Nature, 442, 551-554(2006).
[2] L. Dong, A. K. Agarwal, D. J. Beebe, H. Jiang. Variable-focus liquid microlenses and microlens arrays actuated by thermoresponsive hydrogels. Adv. Mater., 19, 401-405(2007).
[3] S. Colburn, A. Zhan, A. Majumdar. Varifocal zoom imaging with large area focal length adjustable metalenses. Optica, 5, 825-831(2018).
[4] J. Lee, Y. Park, S. K. Chung. Multifunctional liquid lens for variable focus and aperture. Sens. Actuators A, 287, 177-184(2019).
[5] D. Zhu, C. Li, X. Zeng, H. Jiang. Tunable-focus microlens arrays on curved surfaces. Appl. Phys. Lett., 96, 081111(2010).
[6] E. Arbabi, A. Arbabi, S. M. Kamali, Y. Horie, M. Faraji-Dana, A. Faraon. MEMS-tunable dielectric metasurface lens. Nat. Commun., 9, 812(2018).
[7] L. Wang, T. Hayakawa, M. Ishikawa. Dielectric-elastomer-based fabrication method for varifocal microlens array. Opt. Express, 25, 31708-31717(2017).
[8] S. Shian, R. M. Diebold, D. R. Clarke. Tunable lenses using transparent dielectric elastomer actuators. Opt. Express, 21, 8669-8676(2013).
[9] Q. Chen, T. Li, Y. Zhu, W. Yu, X. Zhang. Dielectrophoresis-actuated in-plane optofluidic lens with tunability of focal length from negative to positive. Opt. Express, 26, 6532-6541(2018).
[10] W. Kim, H. C. Yang, D. S. Kim. Wide and fast focus-tunable dielectro-optofluidic lens via pinning of the interface of aqueous and dielectric liquids. Opt. Express, 25, 14697-14705(2017).
[11] L. Li, C. Liu, H. Ren, H. Deng, Q.-H. Wang. Annular folded electrowetting liquid lens. Opt. Lett., 40, 1968-1971(2015).
[12] F. Carpi, G. Frediani, S. Turco, D. De Rossi. Bioinspired tunable lens with muscle-like electroactive elastomers. Adv. Funct. Mater., 21, 4152-4158(2011).
[13] L. Maffli, M. Ghilardi, F. Carpi, H. Shea. Ultrafast all-polymer electrically tunable silicone lenses. Adv. Funct. Mater., 25, 1656-1665(2015).
[14] J. Li, C.-J. Kim. Current commercialization status of electrowetting-on-dielectric (EWOD) digital microfluidics. Lab Chip, 20, 1705-1712(2020).
[15] K. Wei, H. Zeng, Y. Zhao. Insect-human hybrid eye (IHHE): an adaptive optofluidic lens combining the structural characteristics of insect and human eyes. Lab Chip, 14, 3594-3602(2014).
[16] Y. Cheng, C. Chen, J. Cao, C. Bao, A. Yang, Q. Hao. Tunable lens using dielectric elastomer sandwiched by transparent conductive liquid. Opt. Lett., 46, 4430-4433(2021).
[17] N. Hasan, H. Kim, C. H. Mastrangelo. Large aperture tunable-focus liquid lens using shape memory alloy spring. Opt. Express, 24, 13334-13342(2016).
[18] M. B. Kumar, J. Jung, H. Park, J. Hahn, M. Choi, J.-H. Bae, H. Kim, J. Park. Compact vari-focal augmented reality display based on ultrathin, polarization-insensitive, and adaptive liquid crystal lens. Opt. Laser Eng., 128, 106006(2020).
[19] H.-C. Lin, Y.-H. Lin. An electrically tunable-focusing liquid crystal lens with a low voltage and simple electrodes. Opt. Express, 20, 2045-2052(2012).
[20] C.-W. Chiu, Y.-C. Lin, P. C.-P. Chao, A. Y.-G. Fuh. Achieving high focusing power for a large-aperture liquid crystal lens with novel hole-and-ring electrodes. Opt. Express, 16, 19277-19284(2008).
[21] Z. Han, S. Colburn, A. Majumdar, K. F. Böhringer. Millimeter-scale focal length tuning with MEMS-integrated meta-optics employing high-throughput fabrication. Sci. Rep., 12, 5385(2022).
[22] W. Zhang, H. Zappe, A. Seifert. Polyacrylate membranes for tunable liquid-filled microlenses. Opt. Eng., 52, 046601(2013).
[23] J. E. M. Whitehead, A. Zhan, S. Colburn, L. Huang, A. Majumdar. Fast extended depth of focus meta-optics for varifocal functionality. Photon. Res., 10, 828-833(2022).
[24] L. Alvarez. Two-element variable-power spherical lens. U.S. patent(1967).
[25] A. W. Lohmann. A new class of varifocal lenses. Appl. Opt., 9, 1669-1671(1970).
[26] S. Barbero. The Alvarez and Lohmann refractive lenses revisited. Opt. Express, 17, 9376-9390(2009).
[27] M. Peloux, L. Berthelot. Optimization of the optical performance of variable-power and astigmatism Alvarez lenses. Appl. Opt., 53, 6670-6681(2014).
[28] A. Wilson, H. Hua. Design and demonstration of a vari-focal optical see-through head-mounted display using freeform Alvarez lenses. Opt. Express, 27, 15627-15637(2019).
[29] Y. Zou, W. Zhang, F. Tian, F. S. Chau, G. Zhou. Development of miniature tunable multi-element Alvarez lenses. IEEE J. Sel. Top. Quantum Electron., 21, 100-107(2015).
[30] Z. Han, S. Colburn, A. Majumdar, K. F. Böhringer. MEMS-actuated metasurface Alvarez lens. Microsyst. Nanoeng., 6, 79(2020).
[31] Y. Zou, G. Zhou, Y. Du, F. S. Chau. Alignment tolerances and optimal design of MEMS-driven Alvarez lenses. J. Opt., 15, 125711(2013).
[32] G. Zhou, H. Yu, F. S. Chau. Microelectromechanically-driven miniature adaptive Alvarez lens. Opt. Express, 21, 1226-1233(2013).
[33] S. Petsch, A. Grewe, L. Köbele, S. Sinzinger, H. Zappe. Ultrathin Alvarez lens system actuated by artificial muscles. Appl. Opt., 55, 2718-2723(2016).
[34] Y. Zou, W. Zhang, F. S. Chau, G. Zhou. Miniature adjustable-focus endoscope with a solid electrically tunable lens. Opt. Express, 23, 20582-20592(2015).
[35] Y. Zou, F. S. Chau, G. Zhou. Ultra-compact optical zoom endoscope using solid tunable lenses. Opt. Express, 25, 20675-20688(2017).
[36] J. Li, Y. Wang, L. Liu, S. Xu, Y. Liu, J. Leng, S. Cai. A biomimetic soft lens controlled by electrooculographic signal. Adv. Funct. Mater., 29, 1903762(2019).
[37] C. Guo, S. Jiang, L. Yang, P. Song, T. Wang, X. Shao, Z. Zhang, M. Murphy, G. Zheng. Deep learning-enabled whole slide imaging (DeepWSI): oil-immersion quality using dry objectives, longer depth of field, higher system throughput, and better functionality. Opt. Express, 29, 39669-39684(2021).
[38] S. Nam, S. Yun, J. W. Yoon, S. Park, S. K. Park, S. Mun, B. Park, K. U. Kyung. A robust soft lens for tunable camera application using dielectric elastomer actuators. Soft Robot, 5, 777-782(2018).
[39] G. Li, X. Chen, F. Zhou, Y. Liang, Y. Xiao, X. Cao, Z. Zhang, M. Zhang, B. Wu, S. Yin, Y. Xu, H. Fan, Z. Chen, W. Song, W. Yang, B. Pan, J. Hou, W. Zou, S. He, X. Yang, G. Mao, Z. Jia, H. Zhou, T. Li, S. Qu, Z. Xu, Z. Huang, Y. Luo, T. Xie, J. Gu, S. Zhu, W. Yang. Self-powered soft robot in the Mariana trench. Nature, 591, 66-71(2021).
[40] A. She, S. Zhang, S. Shian, D. R. Clarke, F. Capasso. Adaptive metalenses with simultaneous electrical control of focal length, astigmatism, and shift. Sci. Adv., 4, 9957(2018).
[41] L. W. Alvarez, W. E. Humphrey. Variable-power lens and system. US patent(1970).
[42] M. Bawart, A. Jesacher, P. Zelger, S. Bernet, M. Ritsch-Marte. Modified Alvarez lens for high-speed focusing. Opt. Express, 25, 29847-29855(2017).
[43] S. Y. Kang, M. Duocastella, C. B. Arnold. Variable optical elements for fast focus control. Nat. Photonics, 14, 533-542(2020).
[44] H. Ren, S.-T. Wu. Adaptive lenses based on soft electroactive materials. Appl. Sci., 8, 1085(2018).
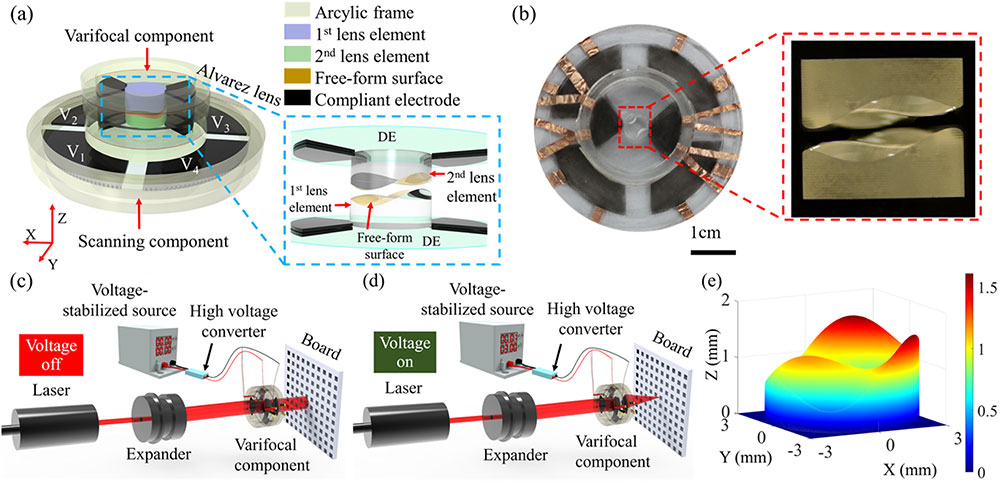
Set citation alerts for the article
Please enter your email address