
- Photonics Research
- Vol. 10, Issue 4, 870 (2022)
Abstract
1. INTRODUCTION
An optical frequency comb is a coherent light source that has applications across a multitude of areas including frequency generation and synthesis [1–4], imaging and sensing [5–8], light detection and ranging [9,10], parallel optical communication and computation [11,12], and quantum information processing [13,14]. The last decade has seen the development of on-chip comb sources implemented in micro-resonators (i.e., microcombs) featuring low power consumption, compact form, and excellent phase stability [15–19]. So far, microcombs have been realized in a variety of integrated photonic platforms such as silicon [20,21], silica [22,23], aluminum nitride [24,25], silicon nitride [26–28], lithium niobate [29–31], and AlGaAs [32,33]. Recently, silicon carbide (SiC) has emerged as a promising photonics material due to its unique material properties, including a wide transparency window (approximately 400–5500 nm), simultaneously possessing strong second- and third-order optical nonlinearities, a relatively high refractive index (nominal index around 2.6 in the telecommunication band), and large thermal conductivity [34]. In addition, SiC hosts a variety of color centers that can be exploited for vital quantum devices such as single-photon sources and quantum memories [35,36]. The combination of these properties renders SiC an attractive platform for photonic applications in both the classical and quantum domains [37–40].
In this work, we are interested in generating broadband microcombs based on the strong third-order nonlinear coefficient (i.e., Kerr combs) offered by SiC [37,41,42]. Due to the direct wafer bonding and polishing technique, thin-film SiC-on-insulator (SiCOI) platforms have been successfully demonstrated for various polytypes of SiC, with the two most common choices being 3C [43–45] and 4H [37,46–49]. To date, microresonators implemented in 4H-SiCOI have achieved the highest quality factors (
Here, we report the first octave-spanning microcomb generation in the 4H-SiCOI platform. This is achieved by engineering the dispersion of the fundamental transverse-electric (
Sign up for Photonics Research TOC. Get the latest issue of Photonics Research delivered right to you!Sign up now
2. SYSTEM DESIGN AND OVERVIEW
The goal of this work is to develop SiC device technologies that enable broadband combs with a telecommunication C-band pump laser (i.e., near 1550 nm). As illustrated in Fig. 1(a), our device platform is based on 4H-SiCOI, where the under-cladding silicon dioxide (
Figure 1.(a) Schematic top view (left) and cross section (right) of the 4H-silicon-carbide-on-insulator platform for frequency comb generation based on compact microring resonators. The sidewall angle (
By computing the resonance frequencies in an eigenmode solver (COMSOL Multiphysics), we extract the group-velocity dispersion (GVD) and higher-order dispersion terms for different ring waveguide widths (RWs). For example, Fig. 1(c) plots the simulated integrated dispersion (
To reach an octave span in the comb spectrum, higher-order dispersion terms need to be included in the design. The integrated dispersion profile displayed in Fig. 1(c) suggests that the comb bandwidth can be further broadened by the so-called dispersive-wave generation at shorter wavelengths of the pump laser for ring widths above 1.7 μm [59]. This is confirmed by numerical simulation based on the Lugiato–Lefever equation (LLE), which takes high-order dispersion terms into consideration [60]. The simulation example provided in Fig. 1(d) is for
3. FABRICATION AND LINEAR CHARACTERIZATION
The device fabrication begins by preparing a 100 mm diameter 4H-SiCOI wafer using the typical bonding and polishing process [47–49]: first, a 2-μm-thick
Figure 2.(a) Optical micrograph of a 36-μm-radius SiC microring with grating input and output. The inset shows the scanning electron micrograph for one section of the ring waveguide, which exhibits smooth sidewalls and
In linear characterization, the light emitted from a telecommunication-band tunable laser (Agilent 81642A with linewidth
4. MICROCOMB GENERATION
To generate frequency combs, the laser output is amplified by an erbium-doped fiber amplifier (EDFA, maximum power up to 2 W) before being coupled to the on-chip waveguide using a grating coupler. For relatively narrowband combs based on
Figure 3.(a) Nonlinear transmission of the
Having successfully generated combs based on
Figure 4.Comb spectra for the
Figure 4 plots the comb spectra of the
5. CONCLUSION
In conclusion, octave-spanning microcombs are demonstrated in the 4H-SiCOI platform for the first time. This outcome is enabled by efforts on two frontiers: first, optimized nanofabrication leads to compact SiC microrings with intrinsic
Acknowledgment
Acknowledgment. The authors acknowledge helpful discussions with Dr. Gordon Keeler and Dr. Tianren Fan, and help with the SiC annealing process from Dr. Xiyuan Lu and Dr. Kartik Srinivasan at NIST. The OSA data in Fig. 4 were obtained with equipment support from Prof. Sheng Shen’s group at CMU as well as Dr. Lijun Ma and Dr. Oliver Slattery at NIST.
References
[1] D. T. Spencer, T. Drake, T. C. Briles, J. Stone, L. C. Sinclair, C. Fredrick, Q. Li, D. Westly, B. R. Ilic, A. Bluestone, N. Volet, T. Komljenovic, L. Chang, S. H. Lee, D. Y. Oh, M.-G. Suh, K. Y. Yang, M. H. P. Pfeiffer, T. J. Kippenberg, E. Norberg, L. Theogarajan, K. Vahala, N. R. Newbury, K. Srinivasan, J. E. Bowers, S. A. Diddams, S. B. Papp. An optical-frequency synthesizer using integrated photonics. Nature, 557, 81-85(2018).
[2] J. Liu, E. Lucas, A. S. Raja, J. He, J. Riemensberger, R. N. Wang, M. Karpov, H. Guo, R. Bouchand, T. J. Kippenberg. Photonic microwave generation in the X- and K-band using integrated soliton microcombs. Nat. Photonics, 14, 486-491(2020).
[3] S.-W. Huang, J. Yang, S.-H. Yang, M. Yu, D.-L. Kwong, T. Zelevinsky, M. Jarrahi, C. W. Wong. Globally stable microresonator Turing pattern formation for coherent high-power THz radiation on-chip. Phys. Rev. X, 7, 041002(2017).
[4] S. A. Diddams, K. Vahala, T. Udem. Optical frequency combs: coherently uniting the electromagnetic spectrum. Science, 369, eaay3676(2020).
[5] C. Bao, M.-G. Suh, K. Vahala. Microresonator soliton dual-comb imaging. Optica, 6, 1110-1116(2019).
[6] S. A. Diddams, L. Hollberg, V. Mbele. Molecular fingerprinting with the resolved modes of a femtosecond laser frequency comb. Nature, 445, 627-630(2007).
[7] G. Ycas, F. R. Giorgetta, K. C. Cossel, E. M. Waxman, E. Baumann, N. R. Newbury, I. Coddington. Mid-infrared dual-comb spectroscopy of volatile organic compounds across long open-air paths. Optica, 6, 165-168(2019).
[8] M.-G. Suh, X. Yi, Y.-H. Lai, S. Leifer, I. S. Grudinin, G. Vasisht, E. C. Martin, M. P. Fitzgerald, G. Doppmann, J. Wang, D. Mawet, S. B. Papp, S. A. Diddams, C. Beichman, K. Vahala. Searching for exoplanets using a microresonator astrocomb. Nat. Photonics, 13, 25-30(2019).
[9] M.-G. Suh, K. J. Vahala. Soliton microcomb range measurement. Science, 359, 884-887(2018).
[10] J. Riemensberger, A. Lukashchuk, M. Karpov, W. Weng, E. Lucas, J. Liu, T. J. Kippenberg. Massively parallel coherent laser ranging using a soliton microcomb. Nature, 581, 164-170(2020).
[11] P. Marin-Palomo, J. N. Kemal, M. Karpov, A. Kordts, J. Pfeifle, M. H. P. Pfeiffer, P. Trocha, S. Wolf, V. Brasch, M. H. Anderson, R. Rosenberger, K. Vijayan, W. Freude, T. J. Kippenberg, C. Koos. Microresonator-based solitons for massively parallel coherent optical communications. Nature, 546, 274-279(2017).
[12] J. Feldmann, N. Youngblood, M. Karpov, H. Gehring, X. Li, M. Stappers, M. Le Gallo, X. Fu, A. Lukashchuk, A. S. Raja, J. Liu, C. D. Wright, A. Sebastian, T. J. Kippenberg, W. H. P. Pernice, H. Bhaskaran. Parallel convolutional processing using an integrated photonic tensor core. Nature, 589, 52-58(2021).
[13] Z. Xie, T. Zhong, S. Shrestha, X. Xu, J. Liang, Y.-X. Gong, J. C. Bienfang, A. Restelli, J. H. Shapiro, F. N. C. Wong, C. W. Wong. Harnessing high-dimensional hyperentanglement through a biphoton frequency comb. Nat. Photonics, 9, 536-542(2015).
[14] M. Kues, C. Reimer, J. M. Lukens, W. J. Munro, A. M. Weiner, D. J. Moss, R. Morandotti. Quantum optical microcombs. Nat. Photonics, 13, 170-179(2019).
[15] T. J. Kippenberg, R. Holzwarth, S. A. Diddams. Microresonator-based optical frequency combs. Science, 332, 555-559(2011).
[16] B. Stern, X. Ji, Y. Okawachi, A. L. Gaeta, M. Lipson. Battery-operated integrated frequency comb generator. Nature, 562, 401-405(2018).
[17] C. Xiang, J. Liu, J. Guo, L. Chang, R. N. Wang, W. Weng, J. Peters, W. Xie, Z. Zhang, J. Riemensberger, J. Selvidge, T. J. Kippenberg, J. E. Bowers. Laser soliton microcombs heterogeneously integrated on silicon. Science, 373, 99-103(2021).
[18] B. Shen, L. Chang, J. Liu, H. Wang, Q.-F. Yang, C. Xiang, R. N. Wang, J. He, T. Liu, W. Xie, J. Guo, D. Kinghorn, L. Wu, Q.-X. Ji, T. J. Kippenberg, K. Vahala, J. E. Bowers. Integrated turnkey soliton microcombs. Nature, 582, 365-369(2020).
[19] W. Jin, Q.-F. Yang, L. Chang, B. Shen, H. Wang, M. A. Leal, L. Wu, M. Gao, A. Feshali, M. Paniccia, K. J. Vahala, J. E. Bowers. Hertz-linewidth semiconductor lasers using CMOS-ready ultra-high-
[20] M. Yu, Y. Okawachi, A. G. Griffith, M. Lipson, A. L. Gaeta. Mode-locked mid-infrared frequency combs in a silicon microresonator. Optica, 3, 854-860(2016).
[21] M. Yu, Y. Okawachi, A. G. Griffith, N. Picqué, M. Lipson, A. L. Gaeta. Silicon-chip-based mid-infrared dual-comb spectroscopy. Nat. Commun., 9, 1869(2018).
[22] X. Yi, Q.-F. Yang, K. Y. Yang, M.-G. Suh, K. Vahala. Soliton frequency comb at microwave rates in a high-
[23] X. Jiang, L. Shao, S.-X. Zhang, X. Yi, J. Wiersig, L. Wang, Q. Gong, M. Lončar, L. Yang, Y.-F. Xiao. Chaos-assisted broadband momentum transformation in optical microresonators. Science, 358, 344-347(2017).
[24] H. Weng, J. Liu, A. A. Afridi, J. Li, J. Dai, X. Ma, Y. Zhang, Q. Lu, J. F. Donegan, J. F. Donegan, W. Guo, W. Guo. Directly accessing octave-spanning dissipative Kerr soliton frequency combs in an AlN microresonator. Photon. Res., 9, 1351-1357(2021).
[25] X. Liu, Z. Gong, A. W. Bruch, J. B. Surya, J. Lu, H. X. Tang. Aluminum nitride nanophotonics for beyond-octave soliton microcomb generation and self-referencing. Nat. Commun., 12, 5428(2021).
[26] Y. Okawachi, K. Saha, J. S. Levy, Y. H. Wen, M. Lipson, A. L. Gaeta. Octave-spanning frequency comb generation in a silicon nitride chip. Opt. Lett., 36, 3398-3400(2011).
[27] Q. Li, T. C. Briles, D. A. Westly, T. E. Drake, J. R. Stone, B. R. Ilic, S. A. Diddams, S. B. Papp, K. Srinivasan. Stably accessing octave-spanning microresonator frequency combs in the soliton regime. Optica, 4, 193-203(2017).
[28] M. H. P. Pfeiffer, C. Herkommer, J. Liu, H. Guo, M. Karpov, E. Lucas, M. Zervas, T. J. Kippenberg. Octave-spanning dissipative Kerr soliton frequency combs in Si3N4 microresonators. Optica, 4, 684-691(2017).
[29] M. Zhang, B. Buscaino, C. Wang, A. Shams-Ansari, C. Reimer, R. Zhu, J. M. Kahn, M. Lončar. Broadband electro-optic frequency comb generation in a lithium niobate microring resonator. Nature, 568, 373-377(2019).
[30] Y. He, Q.-F. Yang, J. Ling, R. Luo, H. Liang, M. Li, B. Shen, H. Wang, K. Vahala, Q. Lin. Self-starting bi-chromatic LiNbO3 soliton microcomb. Optica, 6, 1138-1144(2019).
[31] Z. Gong, X. Liu, Y. Xu, H. X. Tang. Near-octave lithium niobate soliton microcomb. Optica, 7, 1275-1278(2020).
[32] M. Pu, L. Ottaviano, E. Semenova, K. Yvind. Efficient frequency comb generation in AlGaAs-on-insulator. Optica, 3, 823-826(2016).
[33] L. Chang, W. Xie, H. Shu, Q.-F. Yang, B. Shen, A. Boes, J. D. Peters, W. Jin, C. Xiang, S. Liu, G. Moille, S.-P. Yu, X. Wang, K. Srinivasan, S. B. Papp, K. Vahala, J. E. Bowers. Ultra-efficient frequency comb generation in AlGaAs-on-insulator microresonators. Nat. Commun., 11, 1331(2020).
[34] G. Harris. Properties of Silicon Carbide(1995).
[35] C. P. Anderson, A. Bourassa, K. C. Miao, G. Wolfowicz, P. J. Mintun, A. L. Crook, H. Abe, J. U. Hassan, N. T. Son, T. Ohshima, D. D. Awschalom. Electrical and optical control of single spins integrated in scalable semiconductor devices. Science, 366, 1225-1230(2019).
[36] G. Wolfowicz, C. P. Anderson, B. Diler, O. G. Poluektov, F. J. Heremans, D. D. Awschalom. Vanadium spin qubits as telecom quantum emitters in silicon carbide. Sci. Adv., 6, eaaz1192(2020).
[37] J. Cardenas, M. Yu, Y. Okawachi, C. B. Poitras, R. K. W. Lau, A. Dutt, A. L. Gaeta, M. Lipson. Optical nonlinearities in high-confinement silicon carbide waveguides. Opt. Lett., 40, 4138-4141(2015).
[38] H. Kraus, V. A. Soltamov, D. Riedel, S. Väth, F. Fuchs, A. Sperlich, P. G. Baranov, V. Dyakonov, G. V. Astakhov. Room-temperature quantum microwave emitters based on spin defects in silicon carbide. Nat. Phys., 10, 157-162(2014).
[39] D. M. Lukin, M. A. Guidry, J. Vučković. Integrated quantum photonics with silicon carbide: challenges and prospects. PRX Quantum, 1, 020102(2020).
[40] X. Wu, T. Fan, A. A. Eftekhar, A. H. Hosseinnia, A. Adibi. High-
[41] H. Sato, M. Abe, I. Shoji, J. Suda, T. Kondo. Accurate measurements of second-order nonlinear optical coefficients of 6H and 4H silicon carbide. J. Opt. Soc. Am. B, 26, 1892-1896(2009).
[42] X. Lu, J. Y. Lee, S. Rogers, Q. Lin. Optical Kerr nonlinearity in a high-
[43] X. Lu, J. Y. Lee, P. X.-L. Feng, Q. Lin. Silicon carbide microdisk resonator. Opt. Lett., 38, 1304-1306(2013).
[44] T. Fan, H. Moradinejad, X. Wu, A. A. Eftekhar, A. Adibi. High-
[45] T. Fan, X. Wu, A. A. Eftekhar, M. Bosi, H. Moradinejad, E. V. Woods, A. Adibi. High-quality integrated microdisk resonators in the visible-to-near-infrared wavelength range on a 3C-silicon carbide-on-insulator platform. Opt. Lett., 45, 153-156(2020).
[46] Y. Zheng, M. Pu, A. Yi, X. Ou, H. Ou. 4H-SiC microring resonators for nonlinear integrated photonics. Opt. Lett., 44, 5784-5787(2019).
[47] B.-S. Song, T. Asano, S. Jeon, H. Kim, C. Chen, D. D. Kang, S. Noda. Ultrahigh-
[48] D. M. Lukin, C. Dory, M. A. Guidry, K. Y. Yang, S. D. Mishra, R. Trivedi, M. Radulaski, S. Sun, D. Vercruysse, G. H. Ahn, J. Vučković. 4H-silicon-carbide-on-insulator for integrated quantum and nonlinear photonics. Nat. Photonics, 14, 330-334(2020).
[49] C. Wang, Z. Fang, A. Yi, B. Yang, Z. Wang, L. Zhou, C. Shen, Y. Zhu, Y. Zhou, R. Bao, Z. Li, Y. Chen, K. Huang, J. Zhang, Y. Cheng, X. Ou. High-
[50] M. A. Guidry, D. M. Lukin, K. Y. Yang, R. Trivedi, J. Vučković. Quantum optics of soliton microcombs(2021).
[51] A. L. Crook, C. P. Anderson, K. C. Miao, A. Bourassa, H. Lee, S. L. Bayliss, D. O. Bracher, X. Zhang, H. Abe, T. Ohshima, E. L. Hu, D. D. Awschalom. Purcell enhancement of a single silicon carbide color center with coherent spin control. Nano Lett., 20, 3427-3434(2020).
[52] M. A. Guidry, K. Y. Yang, D. M. Lukin, A. Markosyan, J. Yang, M. M. Fejer, J. Vučković. Optical parametric oscillation in silicon carbide nanophotonics. Optica, 7, 1139-1142(2020).
[53] X. Lu, J. Y. Lee, S. D. Rogers, Q. Lin. Silicon carbide double-microdisk resonator. Opt. Lett., 44, 4295-4898(2019).
[54] A. Bartels, D. Heinecke, S. A. Diddams. 10-GHz self-referenced optical frequency comb. Science, 326, 681(2009).
[55] Y. Okawachi, M. Yu, B. Desiatov, B. Y. Kim, T. Hansson, M. Lončar, A. L. Gaeta. Chip-based self-referencing using integrated lithium niobate waveguides. Optica, 7, 702-707(2020).
[56] S. Kim, K. Han, C. Wang, J. A. Jaramillo-Villegas, X. Xue, C. Bao, Y. Xuan, D. E. Leaird, A. M. Weiner, M. Qi. Dispersion engineering and frequency comb generation in thin silicon nitride concentric microresonators. Nat. Commun., 8, 372(2017).
[57] Q. Li, M. Davanço, K. Srinivasan. Efficient and low-noise single-photon-level frequency conversion interfaces using silicon nanophotonics. Nat. Photonics, 10, 406-414(2016).
[58] T. Herr, V. Brasch, J. D. Jost, C. Y. Wang, N. M. Kondratiev, M. L. Gorodetsky, T. J. Kippenberg. Temporal solitons in optical microresonators. Nat. Photonics, 8, 145-152(2014).
[59] V. Brasch, M. Geiselmann, T. Herr, G. Lihachev, M. H. P. Pfeiffer, M. L. Gorodetsky, T. J. Kippenberg. Photonic chip-based optical frequency comb using soliton Cherenkov radiation. Science, 351, 357-360(2016).
[60] S. Coen, H. G. Randle, T. Sylvestre, M. Erkintalo. Modeling of octave-spanning Kerr frequency combs using a generalized mean-field Lugiato-Lefever model. Opt. Lett., 38, 37-39(2013).
[61] D. C. Cole, E. S. Lamb, P. Del’Haye, S. A. Diddams, S. B. Papp. Soliton crystals in Kerr resonators. Nat. Photonics, 11, 671-676(2017).
[62] T. Fan, X. Wu, S. R. M. Vangapandu, A. H. Hosseinnia, A. A. Eftekhar, A. Adibi. Racetrack microresonator based electro-optic phase shifters on a 3C silicon-carbide-on-insulator platform. Opt. Lett., 46, 2135-2138(2021).
[64] X. Xue, X. Zheng, B. Zhou. Super-efficient temporal solitons in mutually coupled optical cavities. Nat. Photonics, 13, 616-622(2019).
[65] Y. Hu, M. Yu, B. Buscaino, N. Sinclair, D. Zhu, R. Cheng, A. Shams-Ansari, L. Shao, M. Zhang, J. M. Kahn, M. Loncar. High-efficiency and broadband electro-optic frequency combs enabled by coupled micro-resonators(2021).
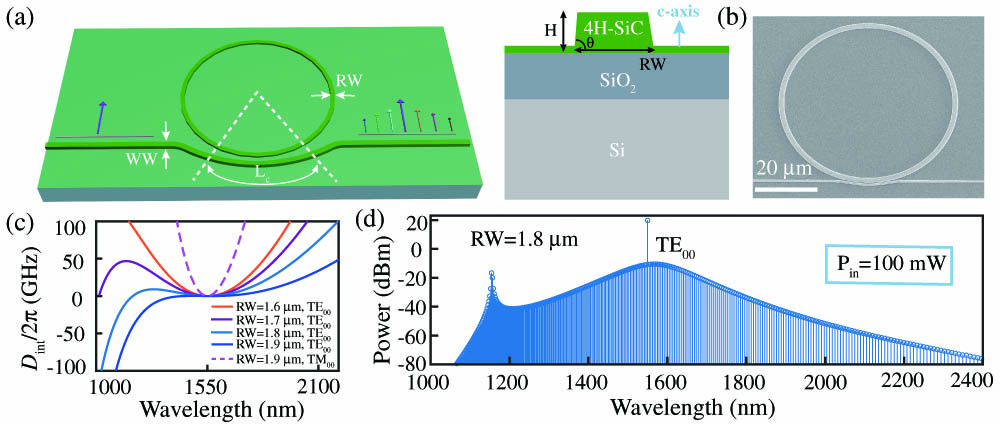
Set citation alerts for the article
Please enter your email address