
- Journal of Semiconductors
- Vol. 41, Issue 3, 032303 (2020)
Abstract
1. Introduction
The generation of laser induced periodic surface structures (LIPSS) represents a particular kind of top down approach to produce highly reproducible nano/microstructures without going for any sophisticated process of lithography. This method is much simpler and cost effective but comes along with a certain degree of randomness in the geometry of the structures. In many cases, however, the aspect of randomness can be well tolerated. Therefore, LIPSS and related structures have been used for various applications such as surface enhanced Raman spectroscopy (SERS), colorization of metals, enhancement of emission efficiency of incandescent light sources, tribology, efficient photoelectron emission, realization of efficient photovoltaic cell and LED, optical memory and controlling hydrophobic properties of materials etc.[
2. Experimental details
2.1. Photocatalytic substrate preparation
Photocatalytically active films were prepared by deposition of TiO2 onto the SiLIPSS using a magnetron sputtering system. The SiLIPSS were prepared with linearly polarized femtosecond (fs) laser pulses at a central wavelength of 800 nm emitted from an amplified Ti:sapphire laser system (Spitfire, Spectra Physics). The duration and repetition rate of the laser pulses were 120 fs and 1 kHz, respectively. The samples were fixed on a computer controlled two-axis translation stage (MFA-CC stages, Newport) in the focal plane of a cylindrical lens (focal length f = 100 mm) for the laser processing. The produced line focus had an extension of 100 × 12 000 μm2. The samples were moved by raster scanning with a velocity of 0.5 mm/s across this line focus to generate LIPSS in an area of 2 × 2 cm2. All the experiments were carried out in air environment. The LIPSS were characterized using a field-emission scanning electron microscope (FESEM, JEOL JSM-6400F). The FESEM image of the SiLIPSS is shown in Fig. 1(a).
Figure 1.(Color online) (a) FESEM images of SiLIPSS (double sided arrow : polarization direction of laser pulses). (b) Related spatial frequency map obtained by 2D-FFT.
These SiLIPSS were generated under optimized working conditions (pulse energy 0.3 mJ, velocity 0.5 mm/s). The orientation of SiLIPSS were found to be perpendicular to the polarization of the laser beam (shown as double sided arrow in Fig. 1(a)). In order to quantitatively estimate the period of these SiLIPSS the two-dimensional fast Fourier transform (2D-FFT) analysis of their FESEM image was done by using ImageJ software. From this 2D-FFT analysis (Fig. 1(b)), the period was estimated to be around 500 nm.
The details of the growth conditions for the TiO2 thin films were discussed in a previous publication[
2.2. Photocatalytic dye decomposition study
A reactor consisting of a UV light source (Philips CLEO Professional 140W-R, UVA-power app. 21 mW/cm2) with a mounted-in magnetic stirrer system was used for photocatalytic induced dye decomposition studies. For this aqueous solution of methylene blue (MB) dye (0.01 mmol/L) was taken as a test medium. The coated samples were put in a solution filled beaker (30 ml) and closed by a quartz plate to prevent solution evaporation. The distance between the test sample and the light source was about 32 cm. Prior to light irradiation, the solution was kept for 30 min in dark condition for complete adsorption-desorption of dyes on the catalyst surface. Thereafter, the samples were irradiated with UV light for the photocatalytic reaction. The whole photoreaction (dark as well light exposure condition) was carried out under continuous slow stirring. To ensure reproducibility and comparability, the experimental conditions were kept the same for all the samples. The MB dye decomposition was measured over a whole irradiation time of four hours by using UV-visible absorption spectroscopy of the solution (Perkin Elmer Lambda 2) comparing the intensities at a wavelength of 664 nm. For comparison, the photocatalysis at the same conditions was carried out with standard Pilkington ActivTM reference glass samples.
3. Results and discussion
3.1. Film structure
The influence of oxygen partial pressure during film deposition on the film structure was investigated by X-ray diffraction (XRD) measurements (Siemens D5000 diffractometer with Cu Kα radiation and a detector scan with an incidence angle of 1°)[
3.2. Photocatalytic activity for high pressure grown TiO2 samples, HPTiO2-SiLIPSS and HPTiO2-NoLIPSS
Fig. 2 shows the UV photocatalytic degradation of MB dye (plot of C/C0 versus time) with high pressure grown TiO2 thin films on Si surface containg LIPSS (HPTiO2-SiLIPSS) and no LIPSS (HPTiO2-NoLIPSS). Here, C0 is the initial concentration of aqueous solution of MB dye and C is its concentration after a certain time of photocatalytic reaction. As can be seen from this figure, the MB dye reduces to about 8% of its initial concentration after 4 h of photocatalytic activity for HPTiO2-SiLIPSS sample whereas it is 30% for HPTiO2-NoLIPSS samples. The corresponding values of MB dye degradation efficiency [(C0 − C)/C0 × 100] are 92% and 70% for HPTiO2-SiLIPSS and HPTiO2-NoLIPSS, respectively. This clearly indicates that the SiLIPSS played an important role in the enhancement of the photocatalytic dye decomposition activity.
Figure 2.(Color online) UV photocatalytic degradation of MB dye (plot of
3.3. Photocatalytic activity for low pressure grown TiO2 samples, LPTiO2-SiLIPSS and LPTiO2-NoLIPSS
Fig. 3 shows the C/C0 versus time plot for MB dye decomposition with low pressure grown TiO2 thin films on Si surface containing LIPSS (LPTiO2-SiLIPSS) and no LIPSS (LPTiO2-NoLIPSS).
Figure 3.(Color online) UV photocatalytic degradation of MB dye (plot of
As can be seen from this figure, the MB dye content reduces to about 25% of its initial concentration after 4 h of photocatalytic activity with the LPTiO2-SiLIPSS sample whereas with LPTiO2-NoLIPSS it is 70%. In this case, the dye decomposition efficiencies are found to be 75% and 30% respectively for LPTiO2-SiLIPSS and LPTiO2-NoLIPSS respectively. So, this again indicates that the SiLIPSS played an important role in enhancement of the photocatalytic dye decomposition activity.
3.4. Evaluation of enhancement of photocatalytic activity in terms of reaction rate constant
The reaction rate constant (k) is a kind of figure of merit parameter in order to quantitatively express the photocatalytic activity of the materials. In order to know further details of the enhancement of the photocatalytic activity due to LIPSS we estimated the reaction rate constants of the various samples by following the standard procedure. In brief, to estimate this parameter first a graph between ln(C0/C) versus photocatalysis time (t) was drawn. Thereafter considering the pseudo-first kinetics, a linear fit line obeying the equation ln(C0/C) = kt was drawn for this graph. The slope of this linear fit line provided us the reaction constant k.
The ln(C0/C) versus photocatalysis time graph for high pressure grown TiO2 thin films, HPTiO2-NoLIPSS and HPTiO2-SiLIPSS are shown in Figs. 4(a) and 4(b), respectively. The quadratic symbols in these figures represent the experimental data and solid line represents their linear fit lines. The reaction rate constants obtained from the slope of the linear fit line of these graphs are given in Table 2. As can be seen from this table, the reaction rate constants of sample HPTiO2-NoLIPSS and HPTiO2-SiLIPSS are 0.26 and 0.54 h–1, respectively.
Figure 4.(Color online) ln(
From the ratio of these reaction rate constants, the enhancement factor of photocatalytic dye decomposition activity of high pressure grown TiO2 thin film in presence of LIPSS is found to be 2.1 (Table 2).
Similarly, the ln(C0/C) versus time graph for low pressure grown TiO2 thin films, LPTiO2-NoLIPSS and LPTiO2-SiLIPSS are shown in Figs. 5(a) and 5(b), respectively.
Figure 5.(Color online) ln(
The reaction rate constants obtained from the slope of the linear fit line of these graphs are given in Table 3.
As can be seen from this table, the reaction rate constants of sample LPTiO2-NoLIPSS and HPTiO2-SiLIPSS are 0.09 and 0.31 h–1, respectively. From the ratio of these reaction rate constant the enhancement factor of photocatalytic dye decomposition activity of low pressure grown TiO2 thin film in presence of LIPSS is found to be 3.3 (Table 3).
It is to note here that the enhancement factor in low pressure TiO2 thin film is greater than high pressure TiO2 thin film. The inferior photocatalytic property of LPTiO2-NoLIPSS is probably the main cause for this.
3.5. Possible cause for enhancement of photocatalytic activity
The LIPSS generally increase roughness and surface area. This phenomenon has already been exploited in frame of applications like increase of friction, wettability etc.[
Figure 6.(Color online) (a) AFM image of the LIPSS on Si (Inset: Selected line for cross-sectional analysis). (b) Cross-sectional analysis across the selected line of (a).
3.6. Comparison of photocatalytic activity of the grown samples with standard reference material
For a reliable evaluation of the enhancement of photocatalytic behavior caused by the newly grown photocatalytic thin film, a comparison to a reference surface is required. One of the best established methods is the calibration with a standard reference thin film like Pilkington ActivTM. The result of such a comparative study is shown in Fig. 7. The reference photocatalytic activity is estimated from the dye decomposition efficiency of various samples after 4 h of photocatalytic activity. As can be seen from this figure, the low pressure grown TiO2 thin film on Si containing no LIPSS (LPTiO2-NoLIPSS sample) shows much inferior photocatalytic activity than Pilkington ActivTM however when it is grown on LIPSS (LPTiO2-SiLIPSS) it can show 1.25 times higher photocatalytic activity than this reference material. The high pressure grown TiO2 thin film on Si containing no LIPSS (HPTiO2-NoLIPSS sample) on the other hand shows almost similar photocatalytic activity as Pilkington ActivTM reference however when it is grown on LIPSS (HPTiO2-SiLIPSS) it can reach about 1.5 times higher photocatalytic activity compared to the reference material.
Figure 7.(Color online) Comparative dye decomposition activity of various samples with respect to standard reference thin film Pilkington ActivTM after 4 h of photocatalysis.
3.7. Significance of present photocatalytic activity enhancement method with respect to the previously reported methods
It has to be noted that a number of methods like doping[
4. Conclusions
To conclude, LIPSS on Si were generated by using a femtosecond laser. The typical period of the LIPSS was 500 nm and their orientation was found to appear perpendicular to the laser polarization. Photocatalytic substrates were prepared by depositing TiO2 thin films on Si surface areas with and without LIPSS. For this purpose, sputtering from a Ti target into two types of oxygen atmospheres were applied. In the first case, the oxygen pressure in the sputtering chamber was chosen to be high (3 × 10–3 mbar) whereas it was by one order of magnitude lower in the second case (2.1 × 10–3 mbar). In the dye decomposition study with MB dye it was found that the presence of LIPSS leads to an enhancement in the photocatalytic reaction rate constant by 2.1 and 3.3 times for high pressure and low pressure grown TiO2 thin films, respectively. The enhancement of surface area in presence of LIPSS is believed to be responsible for the enhanced photocatalytic activity in the present case. Moreover, in a comparative study it was found that the TiO2 thin films grown on LIPSS under low and high oxygen pressure shows a 1.25 and 1.5 times higher photocatalytic activity than the standard reference sample Pilkington ActivTM .
Acknowledgments
Deutsche Forschungsgemeinschaft (DFG), Germany (Grant number GR 1782/12) and Science and Engineering Research Board (SERB), India (Grant number EMR/2015/001175) are acknowledged by R.G. and S.K.D respectively for the financial support. Ms. M. Tischer is acknowledged for technical discussion and support.
References
[1] H W Chang, Y C Tsai, C W Cheng et al. Nanostructured Ag surface fabricated by femtosecond laser for surface-enhanced Raman scattering. J Colloid Interface Sci, 360, 305(2011).
[2]
[3] J Long, P Fan, M Zhong et al. Superhydrophobic and colorful copper surfaces fabricated by picosecond laser induced periodic nanostructures. Appl Surf Sci, 31, 461(2014).
[4] Z Wang, Q Zhao, C Wang. Reduction of friction of metals using laser-induced periodic surface nanostructures. Micromachines, 6, 1606(2015).
[5] A Y Vorobyev, C Guo. Colorizing metals with femtosecond laser pulses. Appl Phys Lett, 92, 041914(2008).
[6] B Dusser, Z Sagan, H Soder et al. Controlled nanostructrures formation by ultra fast laser pulses for color marking. Opt Exp, 18, 2913(2010).
[7] A Y Vorobyev, V S Makin, C Guo. Brighter light sources from black metal: significant increase in emission efficiency of incandescent light sources. Phys Rev Lett, 102, 234301(2009).
[8] T Y Hwang, A Y Vorobyev, C Guo. Surface-plasmon-enhanced photoelectron emission from nanostructure-covered periodic grooves on metals. Phys Rev B, 79, 085425(2009).
[9] R T E Itina, V Vervisch, M Halbwax et al. Study on laser induced periodic structures and photovoltaic application. AIP Conf Proc, 1278, 576(2010).
[10] J T Chen, W C Lai, Y J Kao et al. Laser-induced periodic structures for light extraction efficiency enhancement of GaN-based light emitting diodes. Opt Express, 20, 5689(2012).
[11] S K Das, A Andreev, H Messaoudi et al. Highly periodic laser-induced nanostructures on thin Ti and Cu foils for potential application in laser ion acceleration. J Appl Phys, 119, 13101(2016).
[12] T Baldacchini, J E Carey, M Zhou et al. Superhydrophobic surfaces prepared by microstructuring of silicon using a femtosecond laser. Langmuir, 22, 4917(2006).
[13] Y Shimotsuma, M Sakakura, K Miura et al. Application of femtosecond-laser induced nanostructures in optical memory. J Nanosci Nanotech, 7, 94(2007).
[14] C Yang, W Dong, G Cui et al. Highly-efficient photocatalytic degradation of methylene blue by PoPD-modified TiO2 nanocomposites due to photosensitization-synergetic effect of TiO2 with PoPD. Sci Rep, 7, 3973(2017).
[15] N Julkapli, S Bagheri, S B A Hamid. Recent advances in heterogeneous photocatalytic decolorization of synthetic dyes. Sci World J, 692307(2014).
[16] T Tölke, A Heft, A Pfuch. Photocatalytically active multi-layer systems with enhanced transmission. Thin Solid Films, 516, 4578(2008).
[17] T Tölke, A Kriltz, A Rechtenbach. The influence of pressure on the structure and the self-cleaning properties of sputter deposited TiO2 layers. Thin Solid Films, 518, 4242(2010).
[18] E Granados, M M Calderon, J Krzywinski et al. Enhancement of surface area and wettability properties of boron doped diamond by femtosecond laser-induced periodic surface structuring. Opt Mat Exp, 7, 3389(2017).
[19] R Kuladeep, C Sahoo, D N Rao. Direct writing of continuous and discontinuous sub-wavelength periodic surface structures on single-crystalline silicon using femtosecond laser. Appl Phys Lett, 104, 222103(2014).
[20] V Diesen, C W Dunnill, I P Parkin et al. Silver enhanced TiO2 thin films: photocatalytic characterization using aqueous solutions of tris(hydroxymethyl)aminomethane. Dalton Trans, 43, 344(2014).
[21] S Shuang, R Lv, Z Xie et al. Surface plasmon enhanced photocatalysis of Au/Pt-decorated TiO2 nanopillar arrays. Sci Rep, 6, 26670(2016).
[22] W Cui, D Xue, X Yuan et al. Acid-treated TiO2 nanobelt supported platinum nanoparticles for the catalytic oxidation of formaldehyde at ambient conditions. Appl Surf Sci, 411, 105(2017).
[23] J Chen, W Wang, W Li et al. Roles of crystal surface in Pt-loaded titania for photocatalytic conversion of organic pollutants: a first-principle theoretical calculation. ACS Appl Mater Interfaces, 7, 12671(2015).
[24] M Marelli, C Evangelisti, M V Diamanti et al. TiO2 nanotubes arrays loaded with ligand-free Au nanoparticles: enhancement in photocatalytic activity. ACS Appl Mater Interfaces, 8, 31051(2016).
[25] H L Wang, X H Liu. Preparation of silver nanoparticle loaded mesoporous TiO2 and its photocatalytic property. J Inorg Mater, 31, 555(2016).
[26] H Cheng, C Hsu, Y Chen. Substrate materials and deposition temperature dependent growth characteristics and photocatalytic properties of ALD TiO2 films. J Electrochem Soc, 156, 275(2009).
[27] P Shih, C Huang, T Chen et al. Enhancement on photocatalytic activity of an amorphous titanium oxide film with nano-textured surface by selective-fluorination etching process. Mater Res Bull, 52, 177(2014).
[28] S K Zheng, T M Wang, W C Hao et al. Improvement of photocatalytic activity of TiO2 thin film by Sn ion implantation. Vacuum, 65, 155(2002).
[29] M R Bayati, H M Alipour, S Joshi et al. Thin-film epitaxy and enhancement of photocatalytic activity of anatase/zirconia heterostructures by nanosecond excimer laser treatment. J Phys Chem C, 117, 7138(2013).
[30] P Liu, W Y Li, J B Zhang et al. Photocatalytic activity enhancement of TiO2 porous thin film due to homogeneous surface modification of RuO2. J Mater Res, 26, 1532(2011).
[31] A Álvaro, S Ramírez, A P Próspero et al. Enhanced photocatalytic activity of TiO2 films by modification with polyethylene glycol. Quím Nova, 35, 1931(2012).
[32] J Liu, J Zhang. Photocatalytic activity enhancement of TiO2 nanocrystalline thin film with surface modification of poly-3-hexylthiophene by in situ polymerization. J Mater Res, 31, 1448(2016).
[33] R M Cámara, E Crespo, R Portela et al. Enhanced photocatalytic activity of TiO2 thin films on plasma-pretreated organic polymers. Catal Today, 230, 145(2014).
[34] H E Cheng, C H Hung, I S Yu et al. Strongly enhancing photocatalytic activity of TiO2 thin films by multi-heterojunction technique. Catalysts, 8, 440(2018).
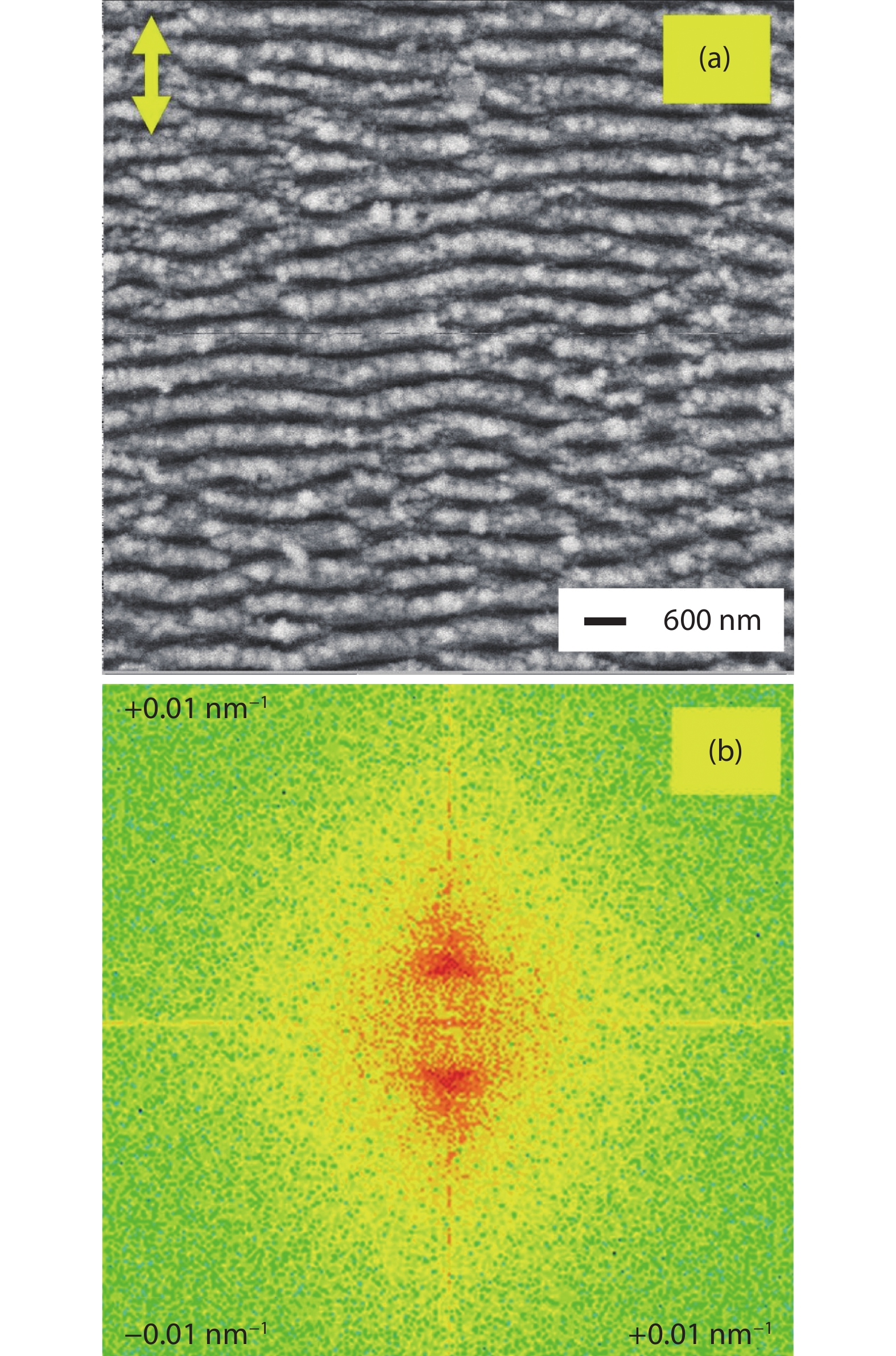
Set citation alerts for the article
Please enter your email address