
- Journal of Semiconductors
- Vol. 40, Issue 6, 062005 (2019)
Abstract
1. Introduction
Two-dimensional (2D) materials, such as black phosphorus (BP)[
Both theoretical and experimental studies[
In this work, the Mo1−xWxSe2 alloys are synthesized on mica, sapphire and SiO2/Si substrates by the chemical vapor deposition (CVD) method. The Mo1−xWxSe2 alloys are first synthesized on the mica substrates by the CVD method. The effects of substrates and interlayer coupling force on the as-grown Mo1−xWxSe2 alloys are then investigated by an atomic force microscope (AFM), Raman spectroscopy and photoluminescence (PL) spectroscopy. The results show that the as-used substrates have negligible effects on the bandgaps of the Mo1−xWxSe2 alloys, while the interlayer coupling effect on the optical bandgaps of Mo1−xWxSe2 alloys is greater than the substrates.
2. Result and discussion
The Mo1−xWxSe2 alloys are prepared on different substrates and are synthesized using the CVD method. The details are introduced in the method section. A schematic of the CVD growing setup is shown in Fig. 1(a). The size of Mo1−xWxSe2 alloys on SiO2/Si substrates as a function of the growing time is illustrated in Fig. 1(b), which reveals that the size of Mo1–xWxSe2 alloys increases with the incremental growth time within 25 min. Raman spectroscopy is a fast and accurate but nondestructive tool to study material structures and properties[
Figure 1.(Color online) The synthesis and characterization of Mo1−
Figs. 2(a)–2(c) show the EDX image of Mo0.8W0.2Se2 alloys on SiO2/Si, sapphire and mica, respectively. These images show that the substrates have no effect on the stoichiometric ratio of the Mo0.8W0.2Se2 alloys. Figs. 2(d)–2(f) show the lattice mismatch images of Mo1−xWxSe2 alloys on mica, SiO2/Si, and sapphire, respectively. The lattice mismatches of Mo1−xWxSe2 alloys with SiO2/Si, sapphire, and mica are about 51 %, 45 % and 52 %, respectively. Figs. 3(a)−3(c) show the optical images observed by the optical microscopy of Mo1−xWxSe2 alloys grown on SiO2/Si, mica, sapphire substrates, respectively. Optical microscopy is the simplest and most effective method to characterize the layered materials. The layer number of the materials can be first identified by the optical microscope. Figs. 3(d)–3(f) show the AFM images of the SiO2/Si, mica, and sapphire, respectively. The root-mean-square (RMS) of the roughness of as-used SiO2/Si, mica and sapphire is 0.128, 0.058, and 0.137 nm, respectively. Thus, mica can provide the smoothest surface among the three substrates for material synthesis.
Figure 2.(Color online) The EDX and lattice mismatch images of as-grown samples on different substrates. (a–c) show the EDX images of Mo0.8W0.2Se2 alloys grown on SiO2/Si, sapphire, and mica, respectively. (d–f) show the lattice mismatch images of Mo1−
Figure 3.(Color online) The optical images of the as-grown Mo1−
PL spectroscopy is a direct method to characterize the optical bandgaps of 2D materials. Fig. 4(a) shows the PL spectra of WSe2, Mo1−xWxSe2 and MoSe2on SiO2/Si substrates. The PL spectrum of Mo1−xWxSe2 located at 1543 meV is in the range between that of WSe2 located at 1592 meV and MoSe2 located at 1510 meV. This result further demonstrates the as-grown samples are alloys. Fig. 4(b) show the PL spectra of monolayer Mo1−xWxSe2 alloys grown on SiO2/Si, mica and sapphire substrates. The changes of PL spectra of Mo1−xWxSe2 alloys on these three substrates are merely about several meV, which demonstrates that the substrates have negligible effects on the optical bandgaps of as-grown Mo1−xWxSe2 alloys. This finding provides an instructive idea for the choice of substrates to grow the alloys by CVD method. Figs. 4(c)–4(e) show the PL spectra of Mo1−xWxSe2 alloys with different layers on sapphire, mica and SiO2/Si, respectively. The bandgaps redshift as the layer numbers of the samples increase, which demonstrates that the interlayer coupling effects related to the layer numbers have large effects on the optical bandgaps of Mo1−xWxSe2 alloys. Fig. 4(f) show the corresponding thickness-dependent PL spectra of Mo1−xWxSe2 alloys on SiO2/Si, mica and sapphire from Figs. 4(c)–4(e). The peak position variations of Mo1−xWxSe2 alloys with different thickness are larger than the Mo1−xWxSe2 alloys growing on different three substrates. Our results demonstrate that the interlayer coupling effect on the optical bandgaps of Mo1−xWxSe2 alloys is greater than the substrates effect.
Figure 4.(Color online) The PL characterization of the as-grown Mo1−
3. Conclusion
In conclusion, Mo1−xWxSe2 films are synthesized on sapphire, mica and SiO2/Si substrates by the CVD method. The AFM, Raman and PL characterization techniques are used to ascertain the monolayer nature of the as-grown samples. The EDX spectra show the adjustable stoichiometric ratio of the 2D alloy materials synthesized by the CVD method. The TEM results indicate the high quality and homogeneity of the as-grown samples. The Raman and AFM results show the roughness of the substrates have a negligible effect on the E2g1 of the Mo1−xWxSe2 alloys. The PL results indicate that the substrates have negligible effects on the bandgaps of Mo1−xWxSe2 alloys and also show that the interlayer coupling effect related to the layer numbers on the optical bandgaps of Mo1−xWxSe2 alloys is larger than the substrates effect. These findings prove a new way for the future study of the growth dynamics and physical properties of two-dimensional materials.
Acknowledgements
This work was supported by the National Natural Science Foundation of China (Nos. 11504111, 61574060), the Projects of Science and Technology Commission of Shanghai Municipality (Nos. 15JC1401800, 14DZ2260800), the Program for Professor of Special Appointment (Eastern Scholar), the Shanghai Rising-Star Program (No. 17QA1401400), the Young Elite Scientists Sponsorship (YESS) Program by CAST, and the Fundamental Research Funds for the Central Universities.
Appendix A
References
[1] G Zhang, S Huang, A Chaves et al. Infrared fingerprints of few-layer black phosphorus. Nat Commun, 8, 14071(2017).
[2] M Mogi, M Kawamura, R Yoshimi et al. A magnetic heterostructure of topological insulators as a candidate for an axion insulator. Nat Mater, 16, 516(2017).
[3] Y Zhang, T R Chang, B Zhou et al. Direct observation of the transition from indirect to direct bandgap in atomically thin epitaxial MoSe2. Nat Nanotechnol, 9, 111(2014).
[4] S Bertolazzi, M Gobbi, Y Zhao et al. Molecular chemistry approaches for tuning the properties of two-dimensional transition metal dichalcogenides. Chem Soc Rev, 47, 6845(2018).
[5] P Manimunda, Y Nakanishi, Y M Jaques et al. Nanoscale deformation and friction characteristics of atomically thin WSe2 and heterostructure using nanoscratch and Raman spectroscopy. 2D Mater, 4, 045005(2017).
[6] C Wang, X Wu, Y Ma et al. Metallic few-layered VSe2 nanosheets: high two-dimensional conductivity for flexible in-plane solid-state supercapacitors. J Mater Chem A, 6, 8299(2018).
[7] S Xiao, P Xiao, X Zhang et al. Atomic-layer soft plasma etching of MoS2. Sci Rep, 6, 19945(2016).
[8] X Zhang, H Nan, S Xiao et al. Shape-uniform, high-quality monolayered MoS2 crystals for gate-tunable photoluminescence. ACS Appl Mater Inter, 9, 42121(2017).
[9] K S Novoselov, A K Geim, S V Morozov et al. Electric field effect in atomically thin carbon flims. Science, 306, 666(2004).
[10] Z Zheng, J Yao, G Yang. Centimeter-scale deposition of Mo0.5W0.5Se2 alloy film for high-performance photodetectors on versatile substrates. ACS Appl Mater Inter, 9, 14920(2017).
[11] J Liu, M Zhong, X Liu et al. Two-dimensional plumbum-doped tin diselenide monolayer transistor with high on/off ratio. Nanotech, 29, 474002(2018).
[12] W Zhou, X Zou, S Najmaei et al. Intrinsic structural defects in monolayer molybdenum disulfide. Nano Lett, 13, 2615(2013).
[13] J Yao, Z Zheng, G Yang. Promoting the performance of layered-material photodetectors by alloy engineering. ACS Appl Mater Inte, 8, 12915(2016).
[14] C P Lu, G Li, J Mao et al. Bandgap, mid-gap states, and gating effects in MoS2. Nano Lett, 14, 4628(2014).
[15] J Kang, S Tongay, J Li et al. Monolayer semiconducting transition metal dichalcogenide alloys: Stability and band bowing. J Appl Phys, 113, 143703(2013).
[16] Q Peng, S De. Tunable band gaps of mono-layer hexagonal BNC heterostructures. Physica E, 44, 1662(2012).
[17] J Ye, B Niu, Y Li et al. Exciton valley dynamics in monolayer Mo1−
[18] C Zhang, S Kc, Y Nie et al. Charge mediated reversible metal−insulator transition in monolayer MoTe2 and W
[19] T Livneh, D O Dumcenco, I Pinkas. Determining alloy composition in Mo
[20] H Li, Q Zhang, X Duan et al. Lateral growth of composition graded atomic layer MoS(2(1−
[21] J Liu, X Liu, Z Chen et al. Tunable Schottky barrier width and enormously enhanced photoresponsivity in Sb doped SnS2 monolayer. Nano Res, 12, 463(2018).
[22] H Li, X Duan, X Wu et al. Growth of alloy MoS(2
[23] X Duan, C Wang, Z Fan et al. Synthesis of WS2
[24] Y Chen, D O Dumcenco, Y Zhu et al. Composition-dependent Raman modes of Mo(1−
[25] D O Dumcenco, H Kobayashi, Z Liu et al. Visualization and quantification of transition metal atomic mixing in Mo1−
[26] J G Song, G H Ryu, S J Lee et al. Controllable synthesis of molybdenum tungsten disulfide alloy for vertically composition-controlled multilayer. Nat Commun, 6, 7817(2015).
[27] D O Dumcenco, K Y Chen, Y P Wang et al. Raman study of 2H-Mo1−
[28] X Zhang, S Xiao, L Shi et al. Large-size Mo1−
[29] T Y Ke, H P Hsu, Y P Wang et al. Temperature dependent piezoreflectance study of Mo1−
[30] M Yarali, H Brahmi, Z Yan et al. Effect of metal doping and vacancies on the thermal conductivity of monolayer molybdenum diselenide. ACS Appl Mater Inter, 10, 4921(2018).
[31] H Xu, X Wu, X Li et al. Properties of graphene-metal contacts probed by Raman spectroscopy. Carbon, 127, 491(2018).
[32] F Liang, H Xu, X Wu et al. Raman spectroscopy characterization of two-dimensional materials. Chin Phys B, 27, 037802(2018).
[33] C Luo, C Wang, X Wu et al. In situ transmission electron microscopy characterization and manipulation of two-dimensional layered materials beyond graphene. Small, 13, 1604259(2017).
[34] J Xia, X Huang, L Z Liu et al. CVD synthesis of large-area, highly crystalline MoSe2 atomic layers on diverse substrates and application to photodetectors. Nanoscale, 6, 8949(2014).
[35] J Huang, L Yang, D Liu et al. Large-area synthesis of monolayer WSe2 on a SiO2/Si substrate and its device applications. Nanoscale, 7, 4193(2015).
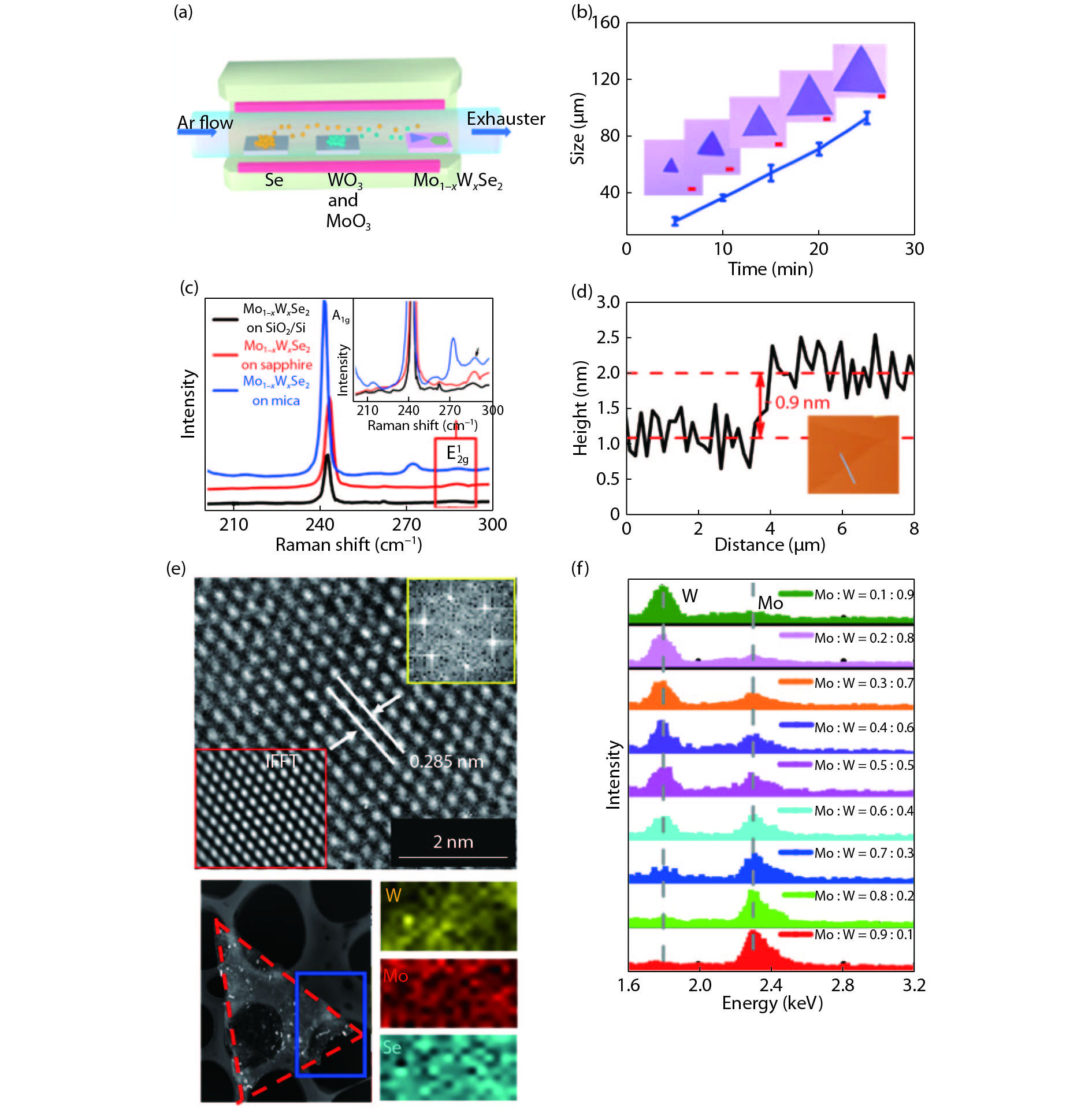
Set citation alerts for the article
Please enter your email address