Fig. 1. Quantum source based on metasurfaces. (
a) Schematic of quantum source based on metasurfaces. (
b) Characterization of three-dimensional, four-dimensional two-photon quantum states. (
c) Manipulating the phase gradient of metasurface to prepare four kinds of bell states. (
d) Characterization of multiphoton quantum source based on metasurface. Figure reproduced from: (a-d) ref.
88, The American Association for the Advancement of Science.
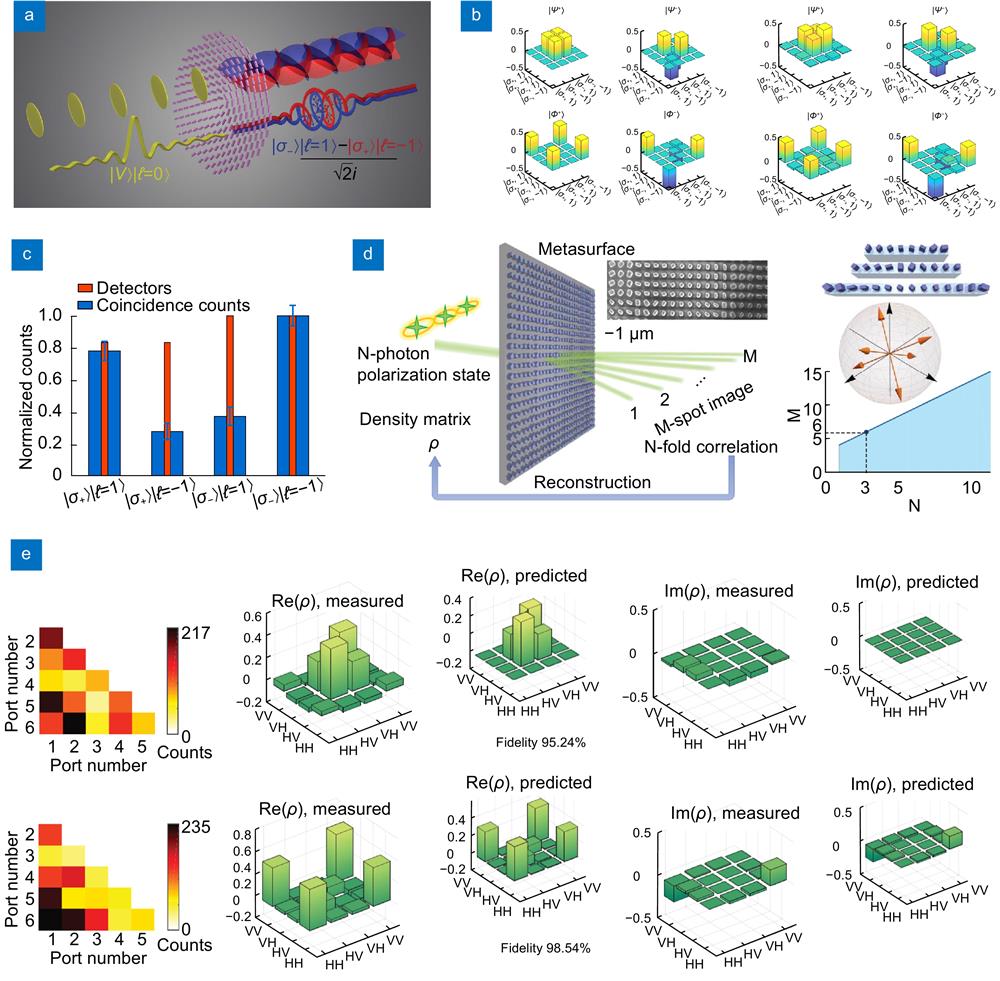
Fig. 2. Manipulation of quantum states based on metasurface. (
a) Employing geometric phase associated with metasurface to make photons with different spin polarization state acquire different orbital angular momentum. (
b) Four bell states of single photon with entanglement of spin angular momentum and orbital angular momentum. (
c) The mutual entanglement between a photon pair. (
d) Left is the schematic of quantum state tomography based on metasurface, top right is three different inter-leaved metasurface, middle-right is the graphical representation of 6 different polarization states, bottom right is relationship between the minimum number of channels required and the number of photons. (
e) The reconstruction of density matrix of two different photon pair state with fidelities 95.24%, 98.54% respectively. Figure reproduced from: (a-c) ref.
89,The American Association for the Advancement of Science; (d-e) ref.
90, The American Association for the Advancement of Science.
Fig. 3. Quantum photonic applications based on metasurfaces. (
a) Absorption of single photon based on metallic metasurfaces,
αand
β represent two photon input channels,
δand
γ represent two photon output channels,
μ andη represent plasmonic input and output channel respectively. (
b) Figure i and ii use signal photon of photon pair to image the metasurface, projective measurements on idle photon is carried out to clearly distinguish triangle and star pattern. Figure iii and iv use photons of mixed state to image metasurface which cannot distinguish the two patterns. (
c) Metasurface is used to entangle and disentangle two photon NOON state. (
d) The position shift and momentum shift of photon wavefunction induced by quantum weak measurement based on metasurfaces. Figure reproduced from: (a) ref.
93, Springer Nature; (b) ref.
96, American Physical Society; (c) ref.
98, Springer Nature; (d) ref.
99, AIP Publishing.
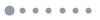
Fig. 4. Quantum vacuum engineering based on metasurfaces. (
a) Based on metasurfaces, the quantum vacuum symmetry of quantum emitter is broken, so that quantum interference between different energy levels of multi-level quantum emitter occurs. (
b) The electromagnetic field radiated by an electric dipole in the
x direction above metasurface can be focused back to the source point along the original path with the maximum efficiency being 81% and the electromagnetic field radiated by an electric dipole in the
y direction has no such effect. (
c) When there is no metasurface, the energy level |
a1 decayed exponentially and the energy level |
a2 occupied 0. When the metasurface exists, the decay rate of the energy level |
a1 decreases, and the energy level |
a2 population firstly increases and then decreases. (
d) Schematic diagram of quantum entanglement of two quantum emitters based on metasurface. (
e) The metasurface enables the electromagnetic field radiated by the source point electric dipole to be oriented to the position of the target electric dipole with highest efficiency 82%. (
f) The concurrence of two quantum emitters varies with the distance between them, the red solid line corresponds to the vacuum condition, and the blue solid line corresponds to the metasurface condition. Figure reproduced from: (a-c) ref.
102, American Physical Society; (d-e) ref.
103, American Physical Society.