Baijun Li, Ran Huang, Xunwei Xu, Adam Miranowicz, Hui Jing, "Nonreciprocal unconventional photon blockade in a spinning optomechanical system," Photonics Res. 7, 630 (2019)

Search by keywords or author
- Photonics Research
- Vol. 7, Issue 6, 630 (2019)
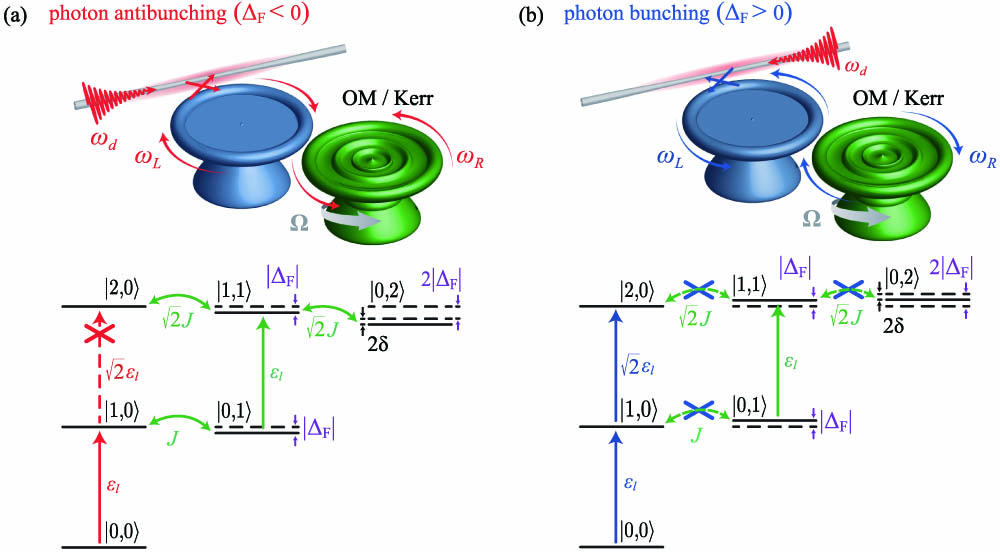
Fig. 1. Nonreciprocal UPB in a coupled-resonator system. Spinning the OM (Kerr-type) resonator results in different Fizeau drag Δ F for the counter-circulating whispering-gallery modes of the resonator. (a) By driving the system from the left-hand side, the direct excitation from state | 1 , 0 ⟩ to state | 2 , 0 ⟩ (red dotted arrow) will be forbidden by destructive quantum interference with the other paths drawn by green arrows, leading to photon antibunching. (b) Photon bunching occurs when the system is driven from the right side, due to lack of complete destructive quantum interference between the indicated levels (drawn by crossed green dotted arrows). Here, δ = g 2 / ω m is the energy shift induced by the OM nonlinearity.
![Correlation function gL(2)(0) versus optical detuning Δ/κ (in units of cavity loss rate κL=κR=κ) with (a) Ω=0 and (b) Ω=12 kHz, which is found numerically (solid curves) and analytically (dotted curve). The PB can be generated (red curves) or suppressed (blue curves) for different driving directions, which can be seen more clearly in panel (c). The other parameters are g/κ=0.63, ωm/κ=10 [91], J/κ=3, T=0.1 mK (case 1), and g/κ=0.1 [28], ωm/κ=30 [92], J/κ=20, T=1 mK (case 2).](/richHtml/prj/2019/7/6/06000630/img_002.jpg)
Fig. 2. Correlation function g L ( 2 ) ( 0 ) versus optical detuning Δ / κ (in units of cavity loss rate κ L = κ R = κ ) with (a) Ω = 0 and (b) Ω = 12 kHz , which is found numerically (solid curves) and analytically (dotted curve). The PB can be generated (red curves) or suppressed (blue curves) for different driving directions, which can be seen more clearly in panel (c). The other parameters are g / κ = 0.63 , ω m / κ = 10 [91], J / κ = 3 , T = 0.1 mK (case 1), and g / κ = 0.1 [28], ω m / κ = 30 [92], J / κ = 20 , T = 1 mK (case 2).
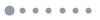
Fig. 3. Correlation function g L ( 2 ) ( 0 ) versus optical detuning Δ / κ (in units of cavity loss rate κ L = κ R = κ ) at various angular velocities Ω upon driving the device from (a) the right-hand side or (b) the left-hand side. The dashed curves show our approximate analytical results, given in Eq. (12 ), whereas the solid curves are our numerical solutions. The other parameters are the same as those in Fig. 2 (case 1).
![Correlation function gL(2)(0) in logarithmic scale [i.e., log10gL(2)(0)] versus (a) radiation-pressure coupling g/κ (in units of cavity loss rate κ=κL=κR) and optical detuning Δ/κ, and (b) coupling strength of the resonators J/κ and radiation-pressure coupling g/κ for optical detuning of Δ/κ=−0.05. The angular velocity is Ω=12 kHz and the white dashed curve corresponds to gL(2)(0)=1. The other parameters are the same as those in Fig. 3.](/Images/icon/loading.gif)
Fig. 4. Correlation function g L ( 2 ) ( 0 ) in logarithmic scale [i.e., log 10 g L ( 2 ) ( 0 ) ] versus (a) radiation-pressure coupling g / κ (in units of cavity loss rate κ = κ L = κ R ) and optical detuning Δ / κ , and (b) coupling strength of the resonators J / κ and radiation-pressure coupling g / κ for optical detuning of Δ / κ = − 0.05 . The angular velocity is Ω = 12 kHz and the white dashed curve corresponds to g L ( 2 ) ( 0 ) = 1 . The other parameters are the same as those in Fig. 3 .
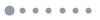
Fig. 5. Correlation function g L ( 2 ) ( 0 ) versus optical detuning Δ / κ (in units of cavity loss rate κ L = κ R = κ ) with varied mean thermal phonon numbers n th for various angular velocities Ω , and the resulting Fizeau shifts Δ F . The other parameters are the same as those in Fig. 4 .
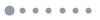
Fig. 6. (a) Correlation function g L ( 2 ) ( 0 ) versus effective temperature T of the environment of the mechanical resonator for three values of Fizeau shift Δ F (Δ F > 0 , Δ F = 0 , and Δ F < 0 ) for optimal values of Δ opt and g opt . The other parameters are set the same as in case 2 in Fig. 2 . Also shown is the correlation function g L ( 2 ) ( 0 ) versus T for various values of (b) spinning frequency, (c) mechanical decay, and (d) cavity decay, assuming the device is driven from the left-hand side and optical detuning is fixed at the optimal values.
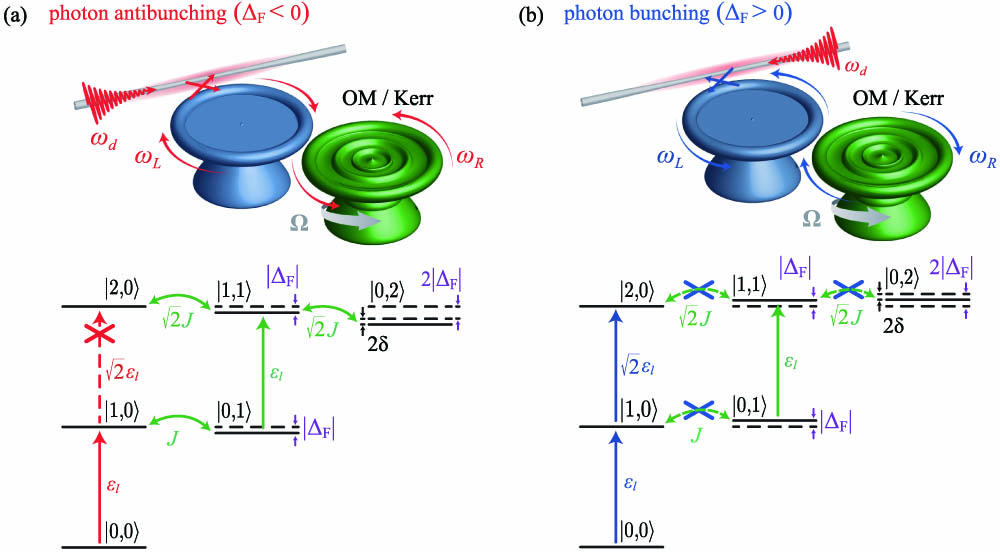
Set citation alerts for the article
Please enter your email address