Mu Yang, Ya Xiao, Ze-Yan Hao, Yu-Wei Liao, Jia-He Cao, Kai Sun, En-Hui Wang, Zheng-Hao Liu, Yutaka Shikano, Jin-Shi Xu, Chuan-Feng Li, Guang-Can Guo, "Entanglement quantification via weak measurements assisted by deep learning," Photonics Res. 12, 712 (2024)

Search by keywords or author
- Photonics Research
- Vol. 12, Issue 4, 712 (2024)
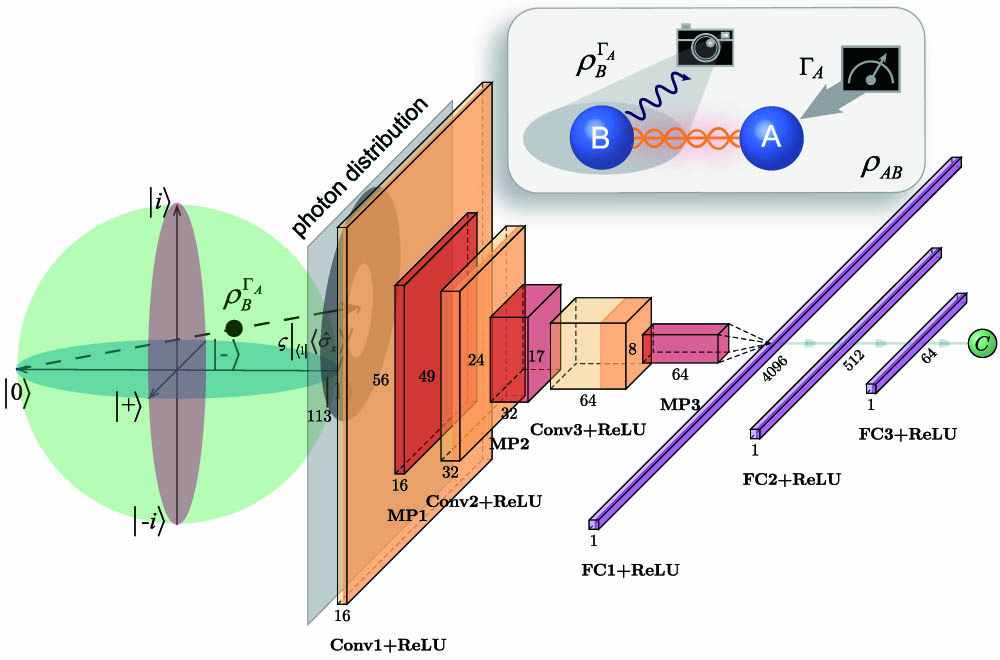
Fig. 1. Theoretical framework and performance of the convolutional neural network (CNN). The weak values ⟨ σ ^ x ⟩ ⟨ 1 | Γ A w and ⟨ σ ^ x ⟩ ⟨ 0 | Γ A w of B on the state of ρ B Γ A (as shown in the Bloch sphere) are encoded in the central position of a Laguerre–Gaussian mode. The projected particle distribution is sent to the CNN to extract the concurrence. The boxes indicate the dimension (width of the box) and number (height of the box) of the feature maps. Convolution layers (Conv) with one stride are shown in a buff color. Activation functions (ReLU) of the convolution layers and full connection layers (FC) are denoted by the orange and purple colors, respectively. The red boxes indicate max-pooling layers (MPs) with two strides, and a small green ball represents the output concurrence. The arrows represent the flow of data. Insert: ρ A B is a two-qubit entangled state. The spatial distribution of B’s conditional state ρ B Γ A is obtained, while a local projective measurement Γ A is performed on A.
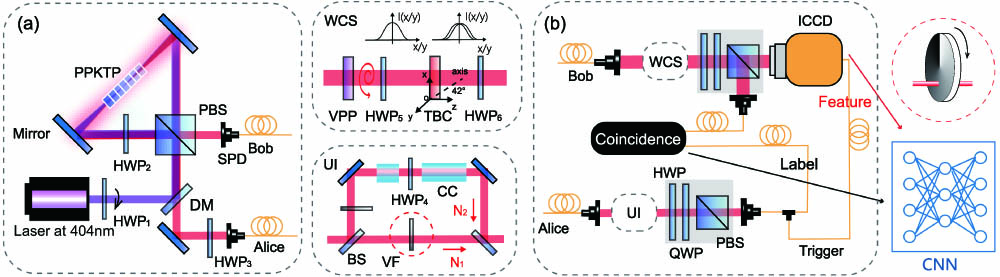
Fig. 2. Experimental setup. (a) A pair of polarization-entangled photons are generated by pumping a type-II PPKTP crystal in a Sagnac interferometer with a 404 nm ultraviolet laser in the preparation stage. A half-wave plate HWP 1 is set in front of the pump laser to rotate the polarizations. The polarizations of the pump light and down-converted photons are exchanged by the dual-wavelength HWP 2 , which is set to 45° in the Sagnac interferometer. HWP 3 is used to change the form of the entangled state and is set to 45°. Bob’s and Alice’s photons pass through a weak measurement system (WM, shown in the gray dotted-line square) and an unbalanced interferometer (UI), respectively. The UI separates the photon into two paths by a beam splitter (BS). There are two sufficiently long calcite crystals (CCs) with the second length being twice larger than that of the first. In-between these crystals, HWP 4 is set to 22.5° in one of the paths. This setup destroys the coherence in the different polarization components. Two variable filters (VFs, in the red dotted line circles) control the relative photon counts between the two arms. In the WM, the photon passes through a vortex phase plate (VPP, l = 2 ) and is shaped into the Laguerre–Gaussian mode. HWP 5 , HWP 6 , and a thin birefringent crystal (TBC) with its axis set to 42° in the x -o -z plane are used to weakly couple the polarizations and momentum of the photon. (b) Quarter-wave plates (QWPs), HWPs, and polarization beam splitters (PBSs) on both sides of Alice and Bob (shown in the gray squares) are used to perform the projective measurements. On Bob’s side, the photons are detected by a single-photon detector (SPD) in the reflected path or by an intensified charge coupled device (ICCD) camera in the transmitted path. The signals detected by the SPD on Alice’s side are sent for coincidence or to trigger the ICCD camera. To train the convolutional neural network (CNN), the concurrence determined from the tomographic data is used as the label and the images recorded by the ICCD camera are used as the features, as indicated by the black and red arrows, respectively.
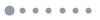
Fig. 3. Conditional states and photon spatial distributions. The numbered dots in the Bloch sphere represent the conditional projective states of Bob. Local photon distribution (I H ) recorded by the ICCD camera with the corresponding concurrence (C act ) is shown in front of the Bloch sphere. The density matrix ρ B Γ A of the number 6 dot and the corresponding input state ρ A B with p = 0.9 and θ = 0.81 are shown; here, the solid and transparent bars represent the experimental and theoretical results, respectively.
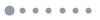
Fig. 4. Experimental results. (a) CNN performance versus epoch. The brown curve represents the MSE value, and the green line represents the PCC between the actual concurrence C act i and the predicted concurrence C pre i . (b) Distribution of predicted concurrences. The blue line represents the optimal curve. (c) Concurrence distributions in the p − θ space. The dots and contour lines represent the experimental and theoretical results, respectively. (d) Logarithm of MSE values of the entangled and separated states versus epoch. (e) CNN fivefold cross-validation performance. The result is averaged five folds. (f) CNN performance versus data size.
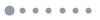
Fig. 5. Value ⟨ σ ^ x ⟩ ⟨ 1 | w of photon B on the state of ρ B Γ A is shown in the Bloch sphere, which is encoded in the photon B’s spatial distribution carrying orbital angular momentum.
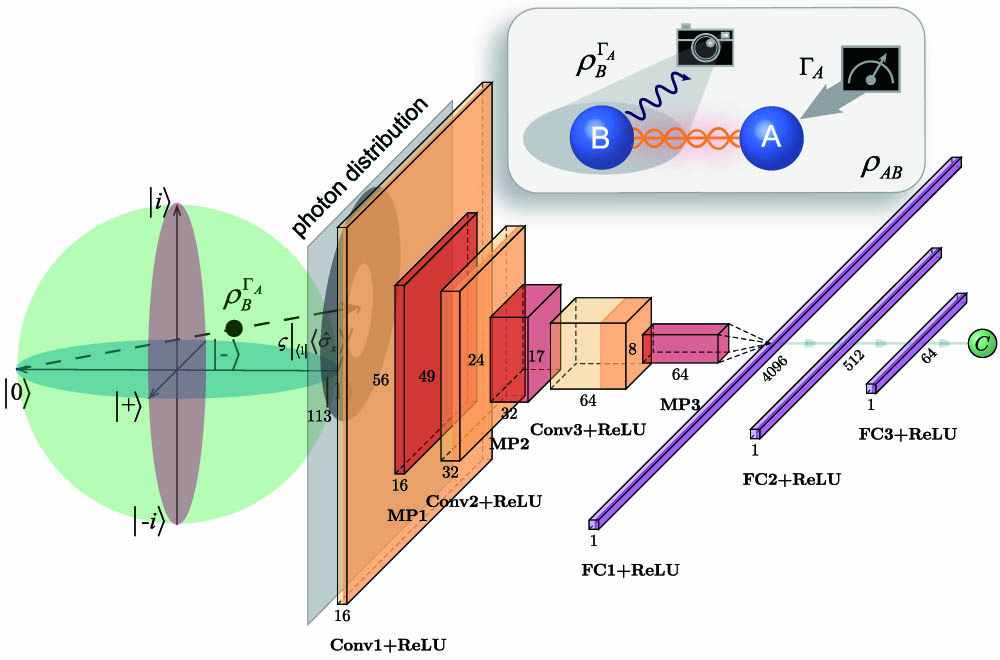
Set citation alerts for the article
Please enter your email address