Jingxin Zhang, Peixing Li, Ray C. C. Cheung, Alex M. H. Wong, Jensen Li, "Generation of time-varying orbital angular momentum beams with space-time-coding digital metasurface," Adv. Photon. 5, 036001 (2023)

Search by keywords or author
- Advanced Photonics
- Vol. 5, Issue 3, 036001 (2023)
![Time-varying OAM beam generation. (a) Time-varying OAM beam with topological charge l(t) varying from 0, 1, 2, 3, 4(−4), −3, −2, and −1 periodically in time, with envelope phase profile [omitting exp(jωt) for brevity] shown at different layers in time. The cyan and red curved surfaces geometrically join up the 0 and π phase on different layers, showing a twist completing one cycle in the clockwise direction defined with winding number w=−1. The beam is generated by a space-time-coding digital metasurface controlled with FPGA. (b) Digital coding scheme of the metasurface with 3-bit coding digits “1” to “8” representing the 0 deg to 315 deg in every 45 deg for the reflection phase profile (in addition to reflection from a perfect metal) at each time instance. The black stars mark the twisted trajectory of zero phase position with coding digit “1.”](/richHtml/ap/2023/5/3/036001/img_001.png)
Fig. 1. Time-varying OAM beam generation. (a) Time-varying OAM beam with topological charge varying from 0, 1, 2, 3, , , , and periodically in time, with envelope phase profile [omitting for brevity] shown at different layers in time. The cyan and red curved surfaces geometrically join up the 0 and phase on different layers, showing a twist completing one cycle in the clockwise direction defined with winding number . The beam is generated by a space-time-coding digital metasurface controlled with FPGA. (b) Digital coding scheme of the metasurface with 3-bit coding digits “1” to “8” representing the 0 deg to 315 deg in every 45 deg for the reflection phase profile (in addition to reflection from a perfect metal) at each time instance. The black stars mark the twisted trajectory of zero phase position with coding digit “1.”
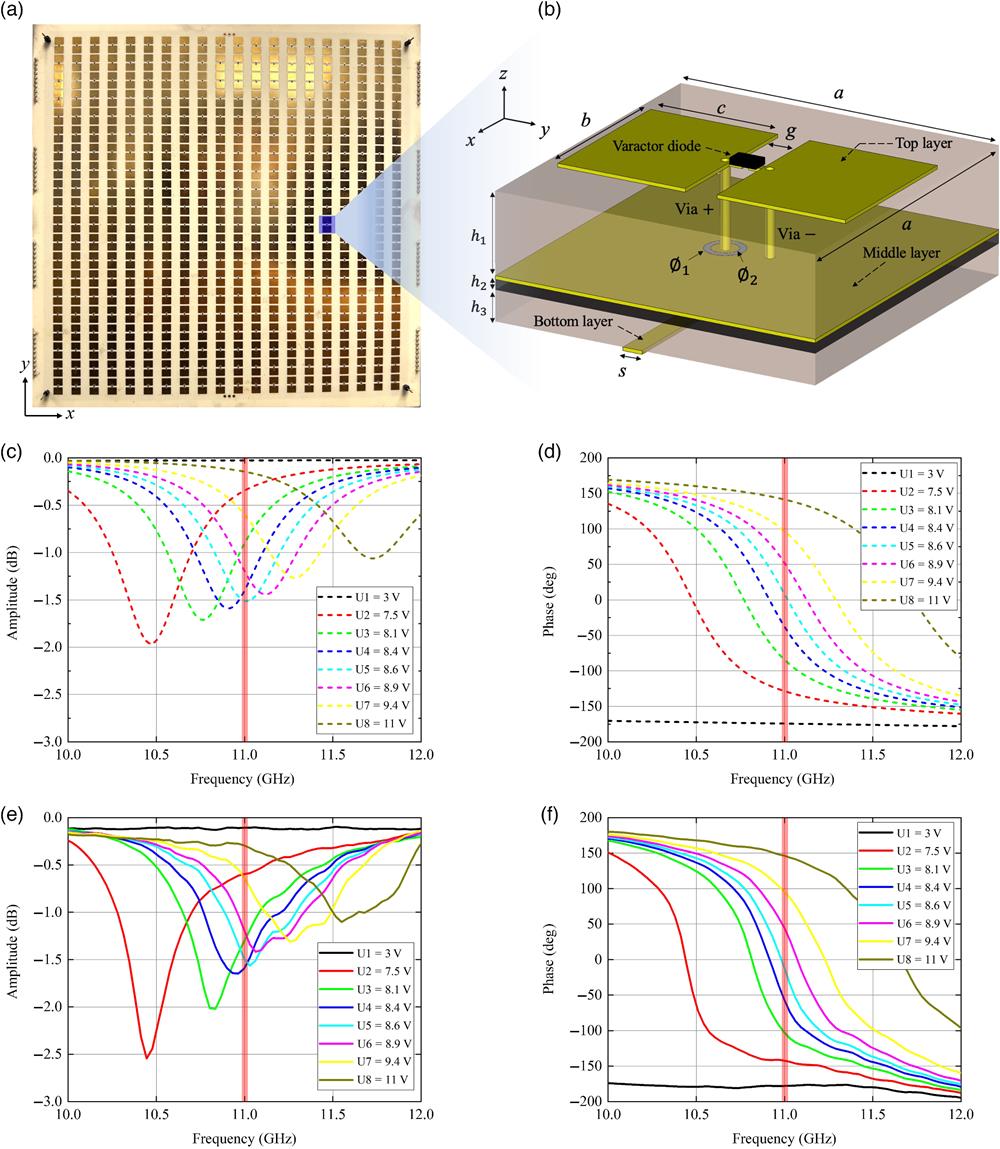
Fig. 2. Metasurface design and reflection responses. (a) Photo of the fabricated space-time-coding digital metasurface. (b) Detailed geometrical parameters of the element structure. (c), (d) Simulated reflection amplitude and phase of the metasurface at different bias voltages, where the vertical orange line indicates the operating frequency at 11 GHz. (e), (f) Measured reflection amplitude and phase of the metasurface at different bias voltages.
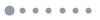
Fig. 3. Dynamic measurement of time-varying OAM field pattern ( ) using the two-probe technique. (a) Experimental scenario of dynamic electric field pattern measurement of the generated time-varying OAM beams. The probe S is movable to scan the field, and the reference probe R is fixed for synchronization processing. (b) Measured near-field amplitude and phase patterns of the time-varying OAM beams at different time instances in one period of . The field patterns consisted of with a range of .
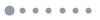
Fig. 4. Spectrum analysis of time-varying OAM. (a) OAM intensity and phase spectrum of the measured time-varying OAM beams at different instants of time. (b) Measured and theoretical OAM mode purity for the dominant . (c) Comparison of measured and theoretical twisting phases for different time instants of dominant .
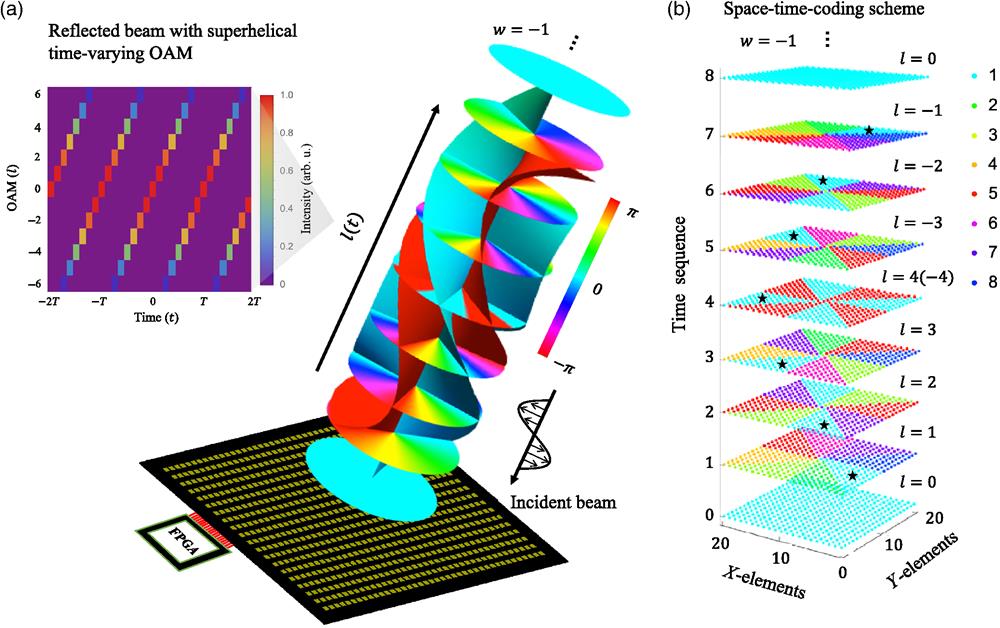
Set citation alerts for the article
Please enter your email address