Guoqiang Zhao, Zheng Deng, Changqing Jin. Advances in new generation diluted magnetic semiconductors with independent spin and charge doping[J]. Journal of Semiconductors, 2019, 40(8): 081505

Search by keywords or author
- Journal of Semiconductors
- Vol. 40, Issue 8, 081505 (2019)
![(Color online) The crystal structure of (a) “111” Li(Zn,Mn)As with zinc blende structure, (b) “122” (Ba,K)(Zn,Mn)2As2 with ThCr2Si2 type structure, (c) (Sr,Na)(Zn,Mn)2As2 with CaAl2Si2 type structure, (d) “1111” (Ba,K)F(Zn,Mn)As with ZrCuSiAs structure. Adoped from Refs. [19, 20, 42, 47].](/richHtml/jos/2019/40/8/081505/img_1.jpg)
Fig. 1. (Color online) The crystal structure of (a) “111” Li(Zn,Mn)As with zinc blende structure, (b) “122” (Ba,K)(Zn,Mn)2As2 with ThCr2Si2 type structure, (c) (Sr,Na)(Zn,Mn)2As2 with CaAl2Si2 type structure, (d) “1111” (Ba,K)F(Zn,Mn)As with ZrCuSiAs structure. Adoped from Refs. [19 , 20 , 42 , 47 ].
![(Color online) Magnetization & transport measurements of Li(Zn,Mn)As. (a) The temperature dependence of M in H = 2 kOe (no difference in FC and ZFC procedures). (b) M at 2 K in various values of external field H from 0 to 20 kOe. The grey symbols show a hysteresis loop in x = 0.03 system plotted for smal field regions (top horizontal axis), which demonstrate a very small coercive field of 30–100 Oe. (c) Resistivity of Li1+yZnAs, showing metallic behavior of Li deficient (y y > 0) compounds. (d) Resistivity of Li 1.1(Zn1–xMnx)As, showing the effect of increasing charge scattering with increasing Mn concentration x. (e) Resistivity of Li1.1(Zn0.9Mn0.1)As in various external field H, which exhibits negative magnetoresistance below TC ~ 50 K. (f) Hall resistivity of Li1.1(Zn0.95Mn0.05)As at 2 K, which exhibits p-type carriers with concentrations of n ~1020 cm–3 together with the anomalous Hall effect due to spontaneous magnetization at H = 0. Adoped from Ref. [19].](/richHtml/jos/2019/40/8/081505/img_2.jpg)
Fig. 2. (Color online) Magnetization & transport measurements of Li(Zn,Mn)As. (a) The temperature dependence of M in H = 2 kOe (no difference in FC and ZFC procedures). (b) M at 2 K in various values of external field H from 0 to 20 kOe. The grey symbols show a hysteresis loop in x = 0.03 system plotted for smal field regions (top horizontal axis), which demonstrate a very small coercive field of 30–100 Oe. (c) Resistivity of Li1+y ZnAs, showing metallic behavior of Li deficient (y < 0) and Li excess ( y > 0) compounds. (d) Resistivity of Li 1.1(Zn1–x Mnx )As, showing the effect of increasing charge scattering with increasing Mn concentration x . (e) Resistivity of Li1.1(Zn0.9Mn0.1)As in various external field H , which exhibits negative magnetoresistance below T C ~ 50 K. (f) Hall resistivity of Li1.1(Zn0.95Mn0.05)As at 2 K, which exhibits p-type carriers with concentrations of n ~1020 cm–3 together with the anomalous Hall effect due to spontaneous magnetization at H = 0. Adoped from Ref. [19 ].
![(Color online) Results of μSR measurements in sintered polycrystalline specimens of Li1.1(Zn0.95Mn0.05)As. (a) Time spectra in zero field that exhibit onset of extra relaxation below T~ 30 K. The solid lines represent fits to the relaxation function for dilute spin systems in zero field for the static case (often used for dilute alloy spin glasses), which exhibits a fast relaxation, plus a non-relaxing paramagnetic component. (b) The relaxation rate α of the signal that exhibits fast relaxation. (c) The volume fraction of the magnetically ordered region, derived from the amplitude of the fast relaxing signal. (d) μSR time spectra in the WTF of 30 Oe. The oscillation amplitude corresponds to the paramagnetic volume fraction. Adoped from Ref. [19].](/Images/icon/loading.gif)
Fig. 3. (Color online) Results of μ SR measurements in sintered polycrystalline specimens of Li1.1(Zn0.95Mn0.05)As. (a) Time spectra in zero field that exhibit onset of extra relaxation below T ~ 30 K. The solid lines represent fits to the relaxation function for dilute spin systems in zero field for the static case (often used for dilute alloy spin glasses), which exhibits a fast relaxation, plus a non-relaxing paramagnetic component. (b) The relaxation rate α of the signal that exhibits fast relaxation. (c) The volume fraction of the magnetically ordered region, derived from the amplitude of the fast relaxing signal. (d) μ SR time spectra in the WTF of 30 Oe. The oscillation amplitude corresponds to the paramagnetic volume fraction. Adoped from Ref. [19 ].
![(Color online) (a) M(H) and ρ(H) in Li1.04Zn0.9Mn0.1P show hysteresis, demonstrating ferromagnetism with small coercive field of about 50 Oe. (b) Magnetoresistivity ρH(T) of Li1.04Zn0.9Mn0.1P at different external fields. Inset shows negative magnetoresistance at low temperature. (c) Arrott plots at various temperatures above and below TC, shows the ferromagnetic transition temperature at 45 K. (d) Negative magnetoresistance at different temperatures. (e) The temperature dependence of M in H = 100 Oe for Li(Zn1–x–0.15CoxMn0.15)As (no difference in ZFC and FC procedures for small coercive fields). (f) Hall resistivity of Li(Zn0.8Co0.1Mn0.1)As at 2 K, which exhibits p-type carriers with concentrations of n ~7.74 × 1019 cm–3 together with the anomalous Hall effect due to spontaneous magnetization at H = 0. Adoped from Refs. [28, 33].](/Images/icon/loading.gif)
Fig. 4. (Color online) (a) M (H ) and ρ (H ) in Li1.04Zn0.9Mn0.1P show hysteresis, demonstrating ferromagnetism with small coercive field of about 50 Oe. (b) Magnetoresistivity ρH (T ) of Li1.04Zn0.9Mn0.1P at different external fields. Inset shows negative magnetoresistance at low temperature. (c) Arrott plots at various temperatures above and below T C, shows the ferromagnetic transition temperature at 45 K. (d) Negative magnetoresistance at different temperatures. (e) The temperature dependence of M in H = 100 Oe for Li(Zn1–x –0.15Cox Mn0.15)As (no difference in ZFC and FC procedures for small coercive fields). (f) Hall resistivity of Li(Zn0.8Co0.1Mn0.1)As at 2 K, which exhibits p-type carriers with concentrations of n ~7.74 × 1019 cm–3 together with the anomalous Hall effect due to spontaneous magnetization at H = 0. Adoped from Refs. [28 , 33 ].
![(Color online) Magnetization & transport measuremets of (Ba,K)(Zn,Mn) 2As2. (a) The tempertature dependence of M in H = 500 Oe for (Ba0.7K0.3)(Zn0.85Mn0.15)2As2 at ZFC and FC procedures with TC 230 K. Inset: The isothermal magnetic hysteresis curve M(H), measured in the external field H up to 7 T. (b) The spontaneous magnetization curve under 5 Oe of (Ba0.7K0.3)(Zn0.85Mn0.15)2As2, showing T3/2 dependence in low temperature expected for a homogeneous ferromagnet. (c) Volume fraction of regions with static magnetic order, estimated by μSR measurements in ZF and weak transverse field (WTF) of 50 G. No hysteresis is seen for WTF measurements with ZF cooling and field cooling in 500 G. Inset: DC magnetization results of the specimens used in μSR measurements. (d) Resistivity of (Ba1–xKx)(Zn1–yMny)2As2 for with several different charge doping levels. (e) Magnetoresistance curve measured in the external field up to 7 T at several selected temperatures, with obvious negative magnetoresistance below TC. Inset: Resistivity curve of (Ba0.7K0.3)(Zn0.85Mn0.15)2As2. (f) Hall effect results from a sintered specimen of (Ba0.85K0.15)(Zn0.90Mn0.10)2As2 at several selected temperatures. A large coercive field is seen at 2 K. Adoped from Refs. [20, 22].](/Images/icon/loading.gif)
Fig. 5. (Color online) Magnetization & transport measuremets of (Ba,K)(Zn,Mn) 2As2. (a) The tempertature dependence of M in H = 500 Oe for (Ba0.7K0.3)(Zn0.85Mn0.15)2As2 at ZFC and FC procedures with T C 230 K. Inset: The isothermal magnetic hysteresis curve M(H), measured in the external field H up to 7 T. (b) The spontaneous magnetization curve under 5 Oe of (Ba0.7K0.3)(Zn0.85Mn0.15)2As2, showing T 3/2 dependence in low temperature expected for a homogeneous ferromagnet. (c) Volume fraction of regions with static magnetic order, estimated by μ SR measurements in ZF and weak transverse field (WTF) of 50 G. No hysteresis is seen for WTF measurements with ZF cooling and field cooling in 500 G. Inset: DC magnetization results of the specimens used in μ SR measurements. (d) Resistivity of (Ba1–x Kx )(Zn1–y Mny )2As2 for with several different charge doping levels. (e) Magnetoresistance curve measured in the external field up to 7 T at several selected temperatures, with obvious negative magnetoresistance below T C. Inset: Resistivity curve of (Ba0.7K0.3)(Zn0.85Mn0.15)2As2. (f) Hall effect results from a sintered specimen of (Ba0.85K0.15)(Zn0.90Mn0.10)2As2 at several selected temperatures. A large coercive field is seen at 2 K. Adoped from Refs. [20 , 22 ].
![(Color online) Magnetization & transport measurements of (Ca,Na)(Zn,Mn) 2As2, (Sr,Na)(Zn,Mn)2As2 and (Sr,Na)(Cd,Mn)2As2. (a) The temperature dependence of M in H = 500 Oe for (Ca0.9Na0.1)(Zn,Mn)2As2 with several different charge doping levels x at ZFC and FC procedures with the highest TC 33 K. (b) The temperature dependence of the volume fraction of regions with static magnetic order, estimated by μSR measurements in ZF, consistent with that of spontaneous magnetization under 5 Oe, which shows T3/2 dependence in low temperature expected for a homogeneous ferromagnet. (c) Curie temperature TC, Weiss temperature θ, effective paramagnetic moment Meff, and saturation moment Msat for (Sr0.9Na0.1)(Zn1–xMnx)2As2 with different spin doping. (d) The temperature dependence of the volume fraction of regions with static magnetic order, estimated by μSR measurements in ZF, consistent with that of spontaneous magnetization under 10 Oe. Inset is the relaxation reats versus temperature, which is consistent with TC. (e) The hysteresis curves of (Sr1–xNax)(Cd1–xMnx)2As2 samples with x = 0.05, 0.1, and 0.125, respectively, at 2 K. (f) Negative magnetoresistance of (Sr0.8Na0.2)(Cd0.8Mn0.2)2As2 at 2 K. Adoped from Refs. [40–42].](/Images/icon/loading.gif)
Fig. 6. (Color online) Magnetization & transport measurements of (Ca,Na)(Zn,Mn) 2As2, (Sr,Na)(Zn,Mn)2As2 and (Sr,Na)(Cd,Mn)2As2. (a) The temperature dependence of M in H = 500 Oe for (Ca0.9Na0.1)(Zn,Mn)2As2 with several different charge doping levels x at ZFC and FC procedures with the highest T C 33 K. (b) The temperature dependence of the volume fraction of regions with static magnetic order, estimated by μ SR measurements in ZF, consistent with that of spontaneous magnetization under 5 Oe, which shows T 3/2 dependence in low temperature expected for a homogeneous ferromagnet. (c) Curie temperature T C, Weiss temperature θ , effective paramagnetic moment M eff, and saturation moment M sat for (Sr0.9Na0.1)(Zn1–x Mnx )2As2 with different spin doping. (d) The temperature dependence of the volume fraction of regions with static magnetic order, estimated by μ SR measurements in ZF, consistent with that of spontaneous magnetization under 10 Oe. Inset is the relaxation reats versus temperature, which is consistent with T C. (e) The hysteresis curves of (Sr1–x Nax )(Cd1–x Mnx )2As2 samples with x = 0.05, 0.1, and 0.125, respectively, at 2 K. (f) Negative magnetoresistance of (Sr0.8Na0.2)(Cd0.8Mn0.2)2As2 at 2 K. Adoped from Refs. [40 –42 ].
![(Color online) (a) Field dependences of magnetization for (La0.95Ca0.05)(Zn0.9Mn0.1)SbO measured at 25 and 100 K. (b) resistivity of (La0.95Ca0.05)(Zn0.925Mn0.075)SbO in various external field H, which exhibits negative magnetoresistance. (c) The temperature dependence of M in H = 500 Oe for (Ba0.8K0.2)F(Zn1–yMny)As with several different charge doping levels y at ZFC and FC procedures with the highest TC 30 K. Inset shows the temperature dependence of the inverse susceptibility for (Ba0.8K0.2)F(Zn0.925Mn0.075)As. (d) The temperature dependence of fast relaxation rate Λ. The error bars represent the estimated standard deviations of the refined parameters. (e) Correlation between TC and the hole concentration for several “111”, “122”, “1111” new types of diluted ferromagnetic semiconductors and typical III–V diluted ferromagnetic semiconductors. Adoped from Refs. [45, 47].](/Images/icon/loading.gif)
Fig. 7. (Color online) (a) Field dependences of magnetization for (La0.95Ca0.05)(Zn0.9Mn0.1)SbO measured at 25 and 100 K. (b) resistivity of (La0.95Ca0.05)(Zn0.925Mn0.075)SbO in various external field H , which exhibits negative magnetoresistance. (c) The temperature dependence of M in H = 500 Oe for (Ba0.8K0.2)F(Zn1–y Mny )As with several different charge doping levels y at ZFC and FC procedures with the highest T C 30 K. Inset shows the temperature dependence of the inverse susceptibility for (Ba0.8K0.2)F(Zn0.925Mn0.075)As. (d) The temperature dependence of fast relaxation rate Λ . The error bars represent the estimated standard deviations of the refined parameters. (e) Correlation between T C and the hole concentration for several “111”, “122”, “1111” new types of diluted ferromagnetic semiconductors and typical III–V diluted ferromagnetic semiconductors. Adoped from Refs. [45 , 47 ].
![(Color online) (a) Mn L2,3-edge XAS spectra of (Ba0.7K0.3)(Zn0.85Mn0.15)2As2 polycrystal. The spectrum is compared with those of (Ga0.922Mn0.078)As, (Ga0.958Mn0.042N), Mn metal, Ba(Fe0.92Mn0.08)2As2, LaMnO3, and MnO.The valence and the local symmetry of the Mn atom are indicated for each compound. (b) & (c) ARPES energy-momentum intensity taken with on- and off-resonance energy photons. (d) & (e) Second derivatives of the on- and off-resonance ARPES spectra. Adoped from Refs. [ 60, 66].](/Images/icon/loading.gif)
Fig. 8. (Color online) (a) Mn L 2,3-edge XAS spectra of (Ba0.7K0.3)(Zn0.85Mn0.15)2As2 polycrystal. The spectrum is compared with those of (Ga0.922Mn0.078)As, (Ga0.958Mn0.042N), Mn metal, Ba(Fe0.92Mn0.08)2As2, LaMnO3, and MnO.The valence and the local symmetry of the Mn atom are indicated for each compound. (b) & (c) ARPES energy-momentum intensity taken with on- and off-resonance energy photons. (d) & (e) Second derivatives of the on- and off-resonance ARPES spectra. Adoped from Refs. [ 60 , 66 ].
![(Color online) (a) The X-ray difraction patterns of (Ba0.904K0.096)(Zn0.805Mn0.195)2As2 at room temperature. The inset shows the crystal structure (right) and its photograph (lef). (b) The tempertature dependence of M in H = 500 Oe for at ZFC and FC procedures in c-axis and ab-plane. (c) The hysteresis curves M(H) measured at 2 K in different axis to exhibit magnetic anisotropy. (d) The temperature dependence of resistivity with current in ab-plane. Inset shows the MR(T) curves in various exterial field strengths. (e) & (f) The anomalous Hall efect Rxy and the magnetoresistance Rxx at several selected temperatures from 2 to 130 K. Adoped from Ref. [67].](/Images/icon/loading.gif)
Fig. 9. (Color online) (a) The X-ray difraction patterns of (Ba0.904K0.096)(Zn0.805Mn0.195)2As2 at room temperature. The inset shows the crystal structure (right) and its photograph (lef). (b) The tempertature dependence of M in H = 500 Oe for at ZFC and FC procedures in c -axis and ab-plane. (c) The hysteresis curves M (H ) measured at 2 K in different axis to exhibit magnetic anisotropy. (d) The temperature dependence of resistivity with current in ab -plane. Inset shows the MR(T) curves in various exterial field strengths. (e) & (f) The anomalous Hall efect Rxy and the magnetoresistance Rxx at several selected temperatures from 2 to 130 K. Adoped from Ref. [67 ].
![(Color online) (a) Sketch of the (Ba0.904K0.096)(Zn0.805Mn0.195)2As2/Pb junctions used for Andreev refection spectroscopy. The inset is the normalization for the diferential conductance G/G0. (b) Normalized diferential conductance G/G0 spectra (red dot) and their fts to the modifed BTK theory (blue line) at selected temperatures at 1.7 K. Adoped from Ref. [67].](/Images/icon/loading.gif)
Fig. 10. (Color online) (a) Sketch of the (Ba0.904K0.096)(Zn0.805Mn0.195)2As2/Pb junctions used for Andreev refection spectroscopy. The inset is the normalization for the diferential conductance G /G 0. (b) Normalized diferential conductance G /G 0 spectra (red dot) and their fts to the modifed BTK theory (blue line) at selected temperatures at 1.7 K. Adoped from Ref. [67 ].
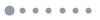
Fig. 11. (colour online) Crystal structures and key physical propertity of (Ba,K)(Zn,Mn)2As2, BaMn2As2, BaZn2As2 and (Ba,K)Fe2As2.
|
Table 1. Some selected properties in new diluted magnetic semiconductors with independent spin & charge doping, superconductors and antiferromagnets.
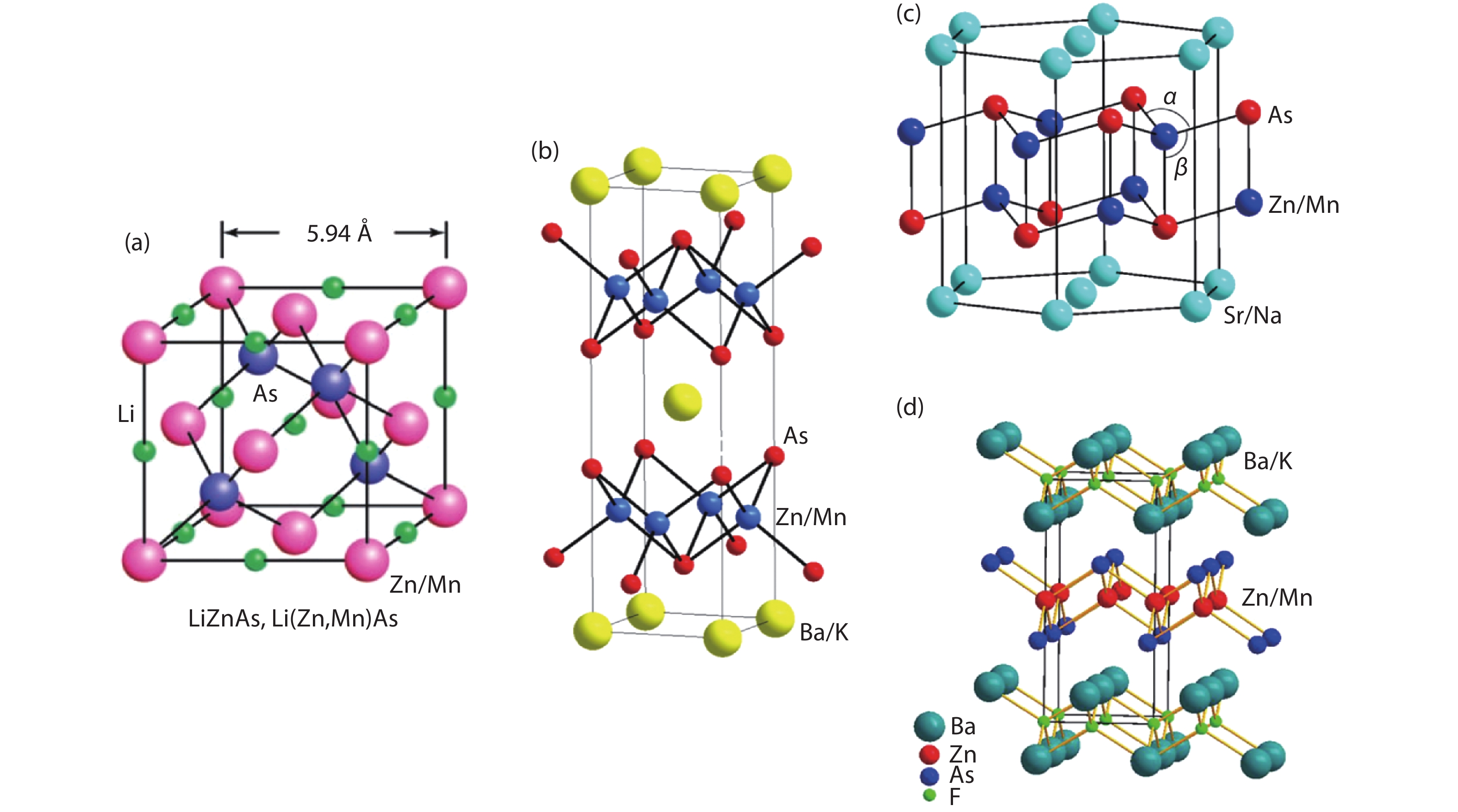
Set citation alerts for the article
Please enter your email address