Daquan Yang, Aiqiang Wang, Jin-Hui Chen, Xiao-Chong Yu, Chuwen Lan, Yuefeng Ji, Yun-Feng Xiao, "Real-time monitoring of hydrogel phase transition in an ultrahigh Q microbubble resonator," Photonics Res. 8, 497 (2020)

Search by keywords or author
- Photonics Research
- Vol. 8, Issue 4, 497 (2020)
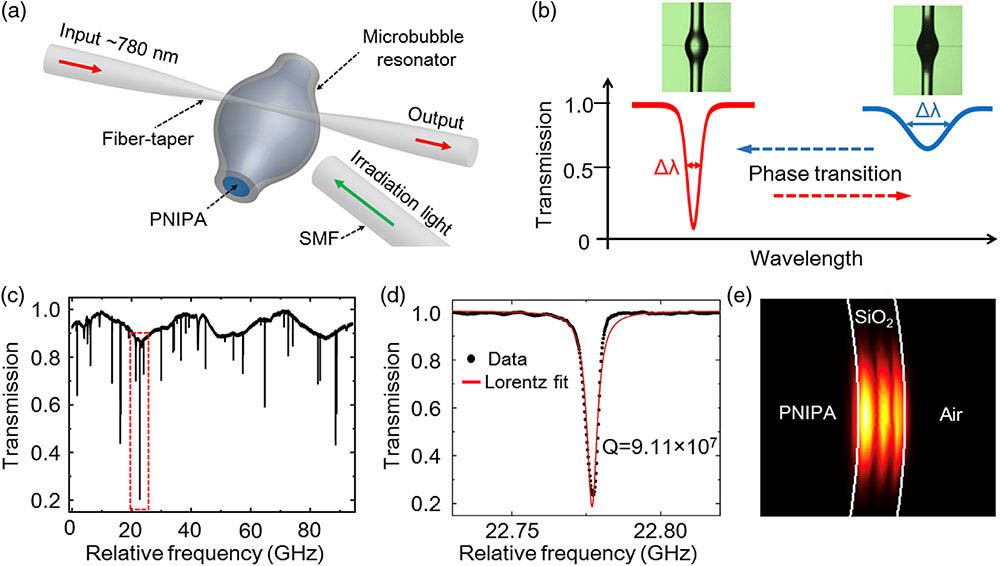
Fig. 1. (a) Schematic of the MBR platform for real-time monitoring of the dynamic reactions of hydrogel phase transition. The thermosensitive phase transition of PNIPA is optically controlled by the irradiation light power (∼ 1550 nm ) from an SMF. (b) Monitoring the phase transition dynamics of the PNIPA solution by tracking the wavelength shift and linewidth broadening of a WGM. Insets, CCD images of the microbubble with the PNIPA solution at hydrophilic and hydrophobic state, respectively. (c) Transmission spectrum of MBR with the PNIPA solution at hydrophilic state. The enlarged view of the red square region is shown in (d). (e) Typical optical field distribution of a WGM in the MBR by finite-element method simulation.
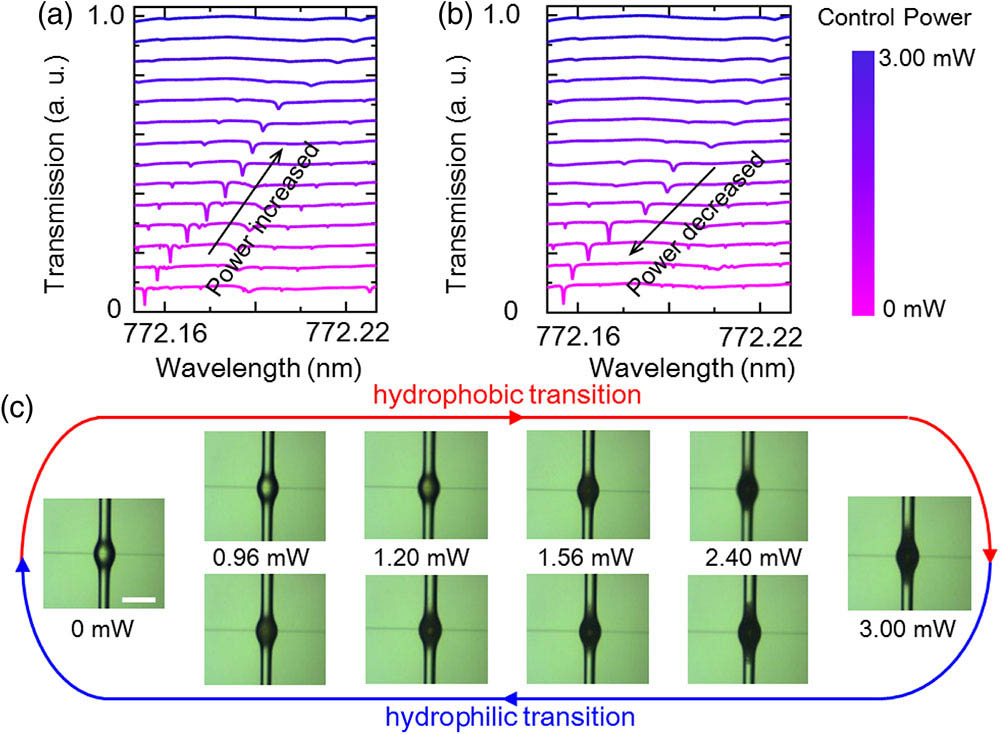
Fig. 2. Transmission evolution of the microbubble with the PNIPA hydrogel when the control power of the irradiation light first (a) increases from 0 to 3.00 mW, and then (b) decreases from 3.00 to 0 mW; (c) CCD images of a cycle of phase-transition process of the PNIPA hydrogel. The microbubble changes from transparent hydrophilic state to opaque hydrophobic state due to the increased scattering. Inset, the scale bar is 125 μm.
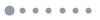
Fig. 3. (a) WGM wavelength shifts and (b) linewidth broadenings as a function of control power of the irradiation light from 0 to 3.00 mW, when the MBRs are filled with air (blue line with triangular marker), DI water (black line with square marker), and PNIPA hydrogel (red line with circular marker). Compared with the result of microbubble cavities filled with air and DI water, note that a hydrophilic to hydrophobic transition process of PNIPA can be clarified as four stages: (i) pure hydrophilic state (0–1.44 mW); (ii) subtransition state (1.44–2.04 mW); (iii) transition state (2.04–2.52 mW); (iv) pure hydrophobic state (> 2.52 mW ).
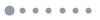
Fig. 4. (a) Real-time WGM resonance wavelength shift and (b) linewidth broadening during the PNIPA hydrogel phase transition (a hydrophilic to hydrophobic transition) monitored by an MBR. The control power of the irradiation light is switched on at ∼ 12.5 s . During the whole phase-transition process, a small blueshift of 8.02 pm in wavelength is first observed within 13.22–15.62 s; then the overall redshift of the resonance wavelength is 39.23 pm, and the maximized linewidth broadening is 3.96 GHz.
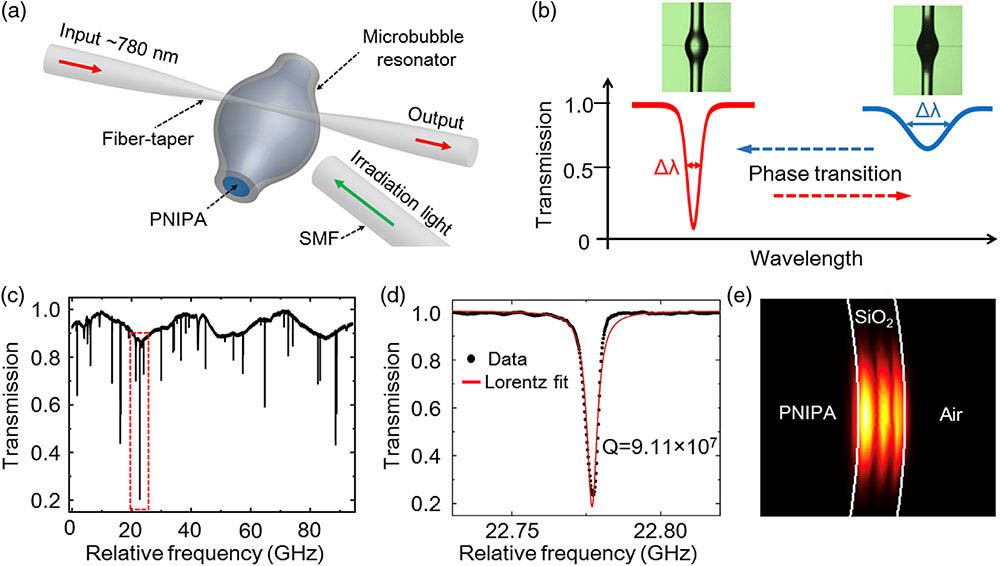
Set citation alerts for the article
Please enter your email address