Hua Pang, Jiahuan Yan, Jie Yang, Shiqi Liu, Yuanyuan Pan, Xiuying Zhang, Bowen Shi, Hao Tang, Jinbo Yang, Qihang Liu, Lianqiang Xu, Yangyang Wang, Jing Lv. Bilayer tellurene–metal interfaces[J]. Journal of Semiconductors, 2019, 40(6): 062003

Search by keywords or author
- Journal of Semiconductors
- Vol. 40, Issue 6, 062003 (2019)
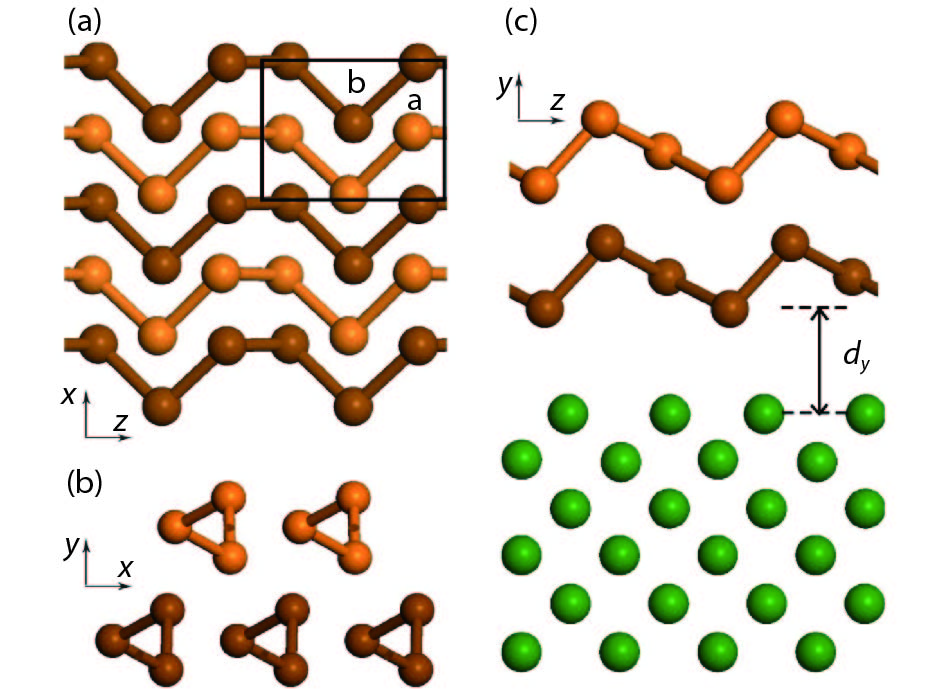
Fig. 1. (Color online) (a) Top-view and (b) side-view of bilayer (BL) tellurene structure. Brown balls represent the contact layer while the orange ones represent the noncontact layer. (c) Schematic diagram of the interface when the BL tellurene atoms contact with metal surface. Green balls stand for the contacting metal atoms.
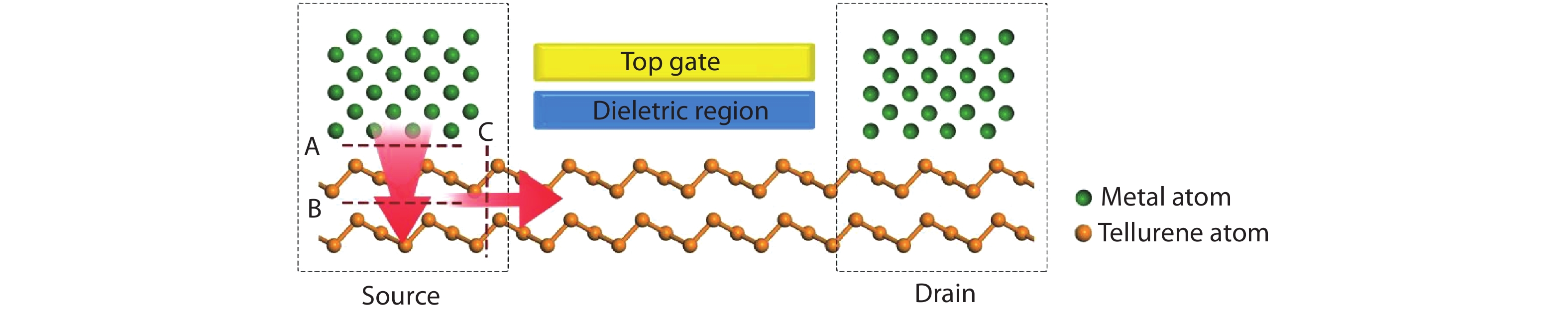
Fig. 2. (Color online) Schematic diagram of the BL tellurene FET. Schottky barriers may arise at the interfaces represented by dashed lines in two directions.
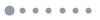
Fig. 3. (Color online) Side-views of the calculated stable BL tellurene–Ag, Al, Ni, Au, Pd, Pt, Cu and graphene contacts. Brown balls are tellurene atoms, while other colored balls are metal and graphene atoms. The diagram of average potential distribution is inset in the black box, where the Fermi level is set to zero with the red dash lines and the tunneling barrier ΔV is shown by the black arrows.
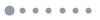
Fig. 4. (Color online) (a) Band structure of the BL tellurene. (b)–(i) Band structure of the BL tellurene-metal systems (projected to the bilayer tellurene). The Fermi level is set at zero and represented by the dashed lines. Gray lines: the band structure of the composite system. The red lines reflect the band structure of the tellurene layer away from the metal surface (the noncontact tellurene layer), the blue ones reflect the band structure of the tellurene layer near the metal surface (the contact tellurene layer). The line width is proportional to its weight.
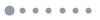
Fig. 5. (Color online) (a) Part density of states (PDOS) of BL tellurene. (b)–(i) PDOS of each orbital for BL tellurene on the metal surface by the band calculations. Solid lines represent for the PDOS of the contact tellurene layer, while dash lines represent for the PDOS of the noncontact tellurene layer.
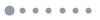
Fig. 6. (Color online) Localized density of states (LDDOS) of the BL tellurene FET devices with metals Al, Ag, Ni, Au, Pd, Pt, Cu and graphene as electrodes (left panel) with a 5-nm channel length as well as the zero-bias transmission spectrum of the FET devices (right panel). Metal-induced gap states at the interfaces are indicated by the black dashed lines, and the Fermi level is represented by white and red dashed lines.
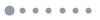
Fig. 7. (Color online) (a) Comparison of the electron and hole SBHs of the BL tellurene FETs obtained by work function approximation (
) and quantum transport simulation
methods in the lateral direction. (b) Lateral SBH as a function of the electrode material’s work function. The blue and pink lines indicate the fitting lines for the SBHs of the electrons for work function approximation and quantum transport simulation, respectively. The pink transparent ellipse represents for the minimal ellipse area that can overcome all the electron SBHs of the bulk metallic electrode cases for the quantum transport calculations. (c) Schematic plot of the Fermi level pinning (FLP) in the BL tellurene transistors.
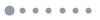
Fig. 8. (Color online) FLP factor (S ) as a function of two-dimensional channel materials’ band gap. R represents for the linear correlation coefficient, where ML black phosphorene and MoS2 are not included.
|
Table 1. Calculated data of the interface with bilayer tellurene on various kinds of metals.
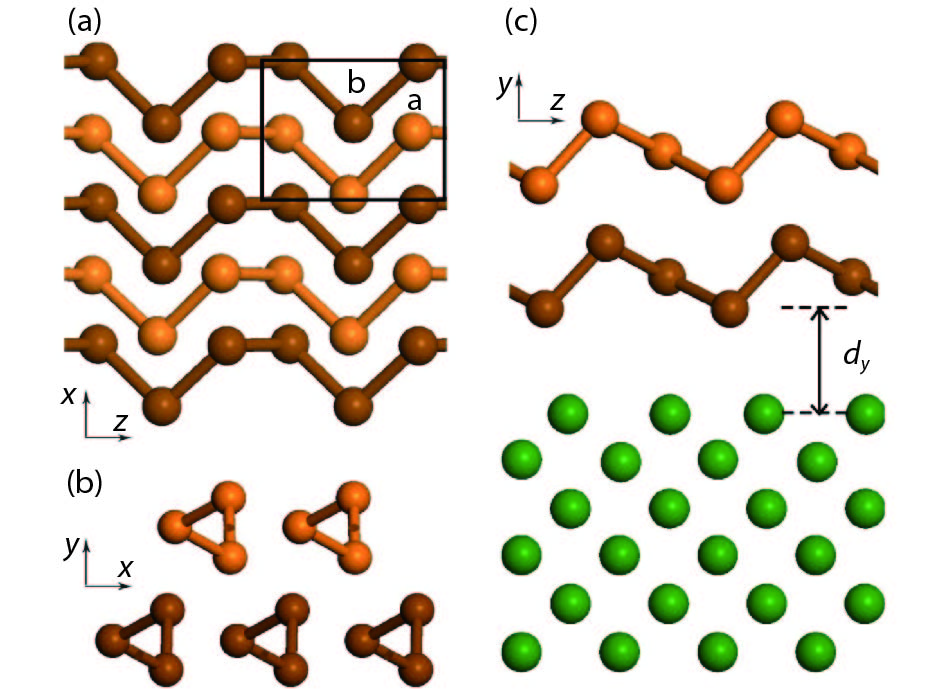
Set citation alerts for the article
Please enter your email address