Abstract
With the epidemic of the coronavirus disease (COVID-19) infection, AlGaN-based ultraviolet-C light emitting diodes (UVC-LEDs) have attracted widespread attention for their sterilization application. However, the sterilization characters of high power integrated light sources (ILSs) haven’t been widely investigated before utilizing in public sanitary security. In this work, by integrating up to 195 UVC-LED chips, high power UVC-LED ILSs with a light output power (LOP) of 1.88 W were demonstrated. The UVC-LED ILSs were verified to have efficient and rapid sterilization capability, which have achieved more than 99.9% inactivation rate of several common pathogenic microorganisms within 1 s. In addition, the corresponding air sterilization module based on them was also demonstrated to kill more than 97% of Staphylococcus albus in the air of 20 m3 confined room within 30 min. This work demonstrates excellent sterilization ability of UVC-LED ILSs with high LOP, revealing great potential of UVC-LEDs in sterilization applications in the future.With the epidemic of the coronavirus disease (COVID-19) infection, AlGaN-based ultraviolet-C light emitting diodes (UVC-LEDs) have attracted widespread attention for their sterilization application. However, the sterilization characters of high power integrated light sources (ILSs) haven’t been widely investigated before utilizing in public sanitary security. In this work, by integrating up to 195 UVC-LED chips, high power UVC-LED ILSs with a light output power (LOP) of 1.88 W were demonstrated. The UVC-LED ILSs were verified to have efficient and rapid sterilization capability, which have achieved more than 99.9% inactivation rate of several common pathogenic microorganisms within 1 s. In addition, the corresponding air sterilization module based on them was also demonstrated to kill more than 97% of Staphylococcus albus in the air of 20 m3 confined room within 30 min. This work demonstrates excellent sterilization ability of UVC-LED ILSs with high LOP, revealing great potential of UVC-LEDs in sterilization applications in the future.Introduction
The global outbreak and epidemic of the coronavirus disease (COVID-19) infection has greatly affected people's health and economic growth, and aroused people's attention to daily sterilization and disinfection. It is known that common disinfection methods could be divided into chemical disinfection and physical disinfection. Chemical disinfectant such as alcohol and strong oxidants can break down the basic protein frame of microorganism, but they are toxic to the human body[1, 2]. Physical disinfection, such as high-temperature treatment and ultraviolet light irradiation, does less damage to human body and has no secondary pollution to the environment. However, the high-temperature treatment will cause damage to the sterilized object itself, which limits its application. Benefitted from the short wavelength (200–280 nm) and high energy, ultraviolet-C (UVC) light has been verified to be able to destroy the chemical bonds inside the deoxyribonucleic acid (DNA) or ribonucleic acid (RNA) of microorganisms, thereby cause the microorganisms to die immediately or fail to reproduce[3-6]. With the advantages of high efficiency, fast speed, and environmental friendliness, UVC radiation disinfection method is gaining more and more attention from both researchers and industrialists.
Mercury lamps are one of the most commonly used UVC light sources, but they have the disadvantages of preheating dependency, large size, and production of ozone which is harmful to the human body[7-9]. What’s more, they contain the toxic element mercury, which will be gradually banned from usage and production by most countries with the implementation of the Minamata Convention on Mercury signed in 2013. AlGaN based UVC light emitting diodes (UVC-LEDs) are believed to be the best alternative for mercury lamps by eliminating the above shortcomings[10-12]. By adjusting the ratio of Al and Ga elements in the active region, single-peak luminescence in the entire UVC band can be achieved[13, 14]. However, they still have lots of scientific issues, and the biggest one is their low wall-plug efficiency (WPE) (mostly < 5%) due to low p-type doping efficiency and low light extraction efficiency [15-19]. Such low WPE makes the light output power (LOP) of the single UVC-LED chip usually less than 100 mW. Studies have confirmed that the inactivation rate of microorganisms by UVC radiation is determined by the UVC radiation dose, which is equal to the irradiance multiplied by the irradiation time[20-22]. The irradiance of UVC-LEDs with low LOP at a certain distance is also low, thus a long irradiation time is required to achieve a high inactivation rate during sterilization, which greatly limits the application of UVC-LEDs in sterilization and disinfection. Fortunately, integrating several to dozens of UVC-LEDs into one light source is a feasible solution to achieve better sterilization effect[23-28]. Lee et al. reported sterilization tests of a homemade sterilizing light source module composed of 40 UVC-LEDs, and it required 30 s to achieve 99% inactivation at an exposure distance of 10 mm[23]. Huang et al. demonstrated a flexible sterilization light source consisting of a square array (3 × 3) of UVC-LEDs with an area of 5 × 5 cm2, which achieved a 95% sterilization inactivation for Escherichia coli in 60 s[24]. However, the integration level and the LOP of the light source are still not high enough, leading to the requirement of long sterilization time.
In this work, different kinds of watt-level LOP UVC-LED integrated light sources (ILSs) with light-emitting wavelength of 275, 265 and 255 nm, respectively, were fabricated for efficient sterilization. By integrating up to 195 UVC-LED chips, the UVC-LED ILS with light-emitting wavelength of 275 nm achieved a LOP of 1.88 W. In addition, the UVC-LED ILSs were demonstrated to show excellent sterilization and disinfection capabilities, which could kill more than 99.9% of Escherichia coli, Staphylococcus aureus and Candida albicans on the surface within 1 s. Besides, an air sterilization module was demonstrated by combining the UVC-LED ILS and a well-designed air tube, which achieved over 97% inactivation of Staphylococcus albus in the air of 20 m3 confined space within 30 min.
Results and discussion
Deep ultraviolet LED integrated light source
The UVC-LED ILSs with package structure of ‘chip on board’ (COB) were designed to achieve high integration, as shown in Fig. 1. The preparation process takes the following steps. Firstly, 195 pieces of UVC-LED chips with size of 20 × 20 mil2 were eutectic soldered on a 1-mm-thick AlN ceramic circuit board by electrical connection of 15 series and 13 parallels. The light-emitting area was set to 3.4 × 3.4 cm2. Detailed information regarding to UVC-LED epitaxial growth and chip fabrication could be found in our previous results[27]. Secondly, the chip array was surrounded by a ceramic dam and subsequently covered by a quartz glass which has high transparency to UVC light. Thirdly, a cooling system consisted of a copper cooling plate with dense fins and a cooling fan with size of 8 × 8 cm2 was mounted on the back of the AlN ceramic circuit board to dissipate heat generated by UVC-LED chips. The power supply of the light source was self-assembled, which could realize a continuous adjustment of the injection current from 0.1 to 1.3 A and display the output voltage value through a digital screen. Taking the above fabrication processes, three UVC-LED ILSs based on three types of chips with light-emitting wavelength of 275, 265 and 255 nm were fabricated, which are named as 275-nm-UVC-LED ILS, 265-nm-UVC-LED ILS and 255-nm-UVC-LED ILS, respectively.
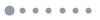
Figure 1.(Color online) Schematic of UVC-LED ILS: (a) assembly diagram, (b) explosive diagram.
The electrical and optical performances of UVC-LED ILSs were collected by an EVERFINE ATA-500UV opto-electronic analyzer. In order to obtain accurate results, the opto-electronic analyzer was calibrated by a standard UVC-LED before measurements. Fig. 2(a) shows the LOP of three UVC-LED ILSs driven under different current injections. It can be seen that the LOP increases linearly with the increase of injected current. When the current increases to a maximum of 1.3 A (equivalent to 100 mA for each chip), the LOP reaches 1.88, 1.43 and 0.99 W for 275-nm-UVC-LED ILS, 265-nm-UVC-LED ILS and 255-nm-UVC-LED ILS, respectively. The difference of LOP between three light sources could be attributed to the wavelength dependent efficiency difference in three kinds of UVC-LED chips. The current–voltage curves are shown in Fig. 2(b), which all exhibit clear diode characteristics. Specifically, with increasing injection current, the voltage rises rapidly at first and then gradually saturates at ~81 V (equivalent to 5.4 V for each chip). The WPEs of three UVC-LED ILSs under different current were calculated and shown in Fig. 2(c). One can see that the 275-nm-UVC-LED ILS has achieved a high WPE of ~3% under 100 mA current injection. However, all the three UVC-LED ILSs suffer from an efficiency droop with increasing injection current as commonly reported[29-32]. The EL spectra of 275-nm-UVC-LED ILS, 265-nm-UVC-LED ILS and 255-nm-UVC-LED ILS presented in Fig. 2(d) exhibits peak wavelengths of 274.8, 265.8 and 255.6 nm, respectively. The full width at half maximum (FWHMs) are estimated to 11.7, 11.3 and 11.4 nm, respectively, indicating the unique light-emitting wavelength uniformity of our UVC-LED chips.
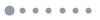
Figure 2.(Color online) Characterization of three UVC-LED ILSs: (a) LOP vs. current, (b) voltage vs. current, (c) WPE vs. current, (d) EL spectra.
Taking the 275-nm-UVC-LED ILS as an example, the irradiance distribution of UVC-LED ILSs has been characterized and studied carefully. Firstly, the irradiance at different distance from the 275-nm-UVC-LED ILS under an injection current of 0.8 A has been measured, as shown in Fig. 3(a). As we can see, the irradiance drops rapidly and then slowly with increasing distance. From the inset, one can generalize that the reciprocal of irradiance is approximately proportional to the square of distance, indicating that irradiance and distance have an inverse square relationship, which is consistent with the spherical diffusion model of light. The irradiance distribution of central axis of the light-emitting area is presented in Fig. 3(b). Here, the center of the light-emitting area is set as the zero point of central axis. We can see that the FWHM of irradiance distribution curve is about 3.3 cm, which is very close to the width of the light-emitting area of 3.4 cm, demonstrating that our UVC-LED ILSs have excellent irradiation uniformity on the light-emitting surface.
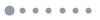
Figure 3.(Color online) (a) Irradiance at different distances from the 275-nm-UVC-LED ILS under an injection current of 0.8 A. The inset shows that the reciprocal of irradiance is approximately proportional to the square of distance. (b) Irradiance distribution of the central axis of light-emitting area (x = 0 at the center of light-emitting area). (c) Temperature change with time after the 275-nm-UVC-LED ILS was turned on. The inset shows the locations for temperature measurements: p1 and p2 are the center and the edge of the quartz glass on light-emitting area, respectively, and p3 is located on AlN ceramic substrate and close to the light-emitting area. (d) Irradiance at a distance of 5 cm from the light-emitting surface of the 275-nm-UVC-LED ILS under different current injection.
It is known that UVC-LEDs generate a great amount of heat during normal operation due to their low WPE, so it’s very important to achieve good heat dissipation of UVC-LEDs, especially high-power UVC-LED ILSs. In order to evaluate the heat distribution of our UVC-LED ILSs, the time-dependent temperature change of the 275-nm-UVC-LED ILS in continuous working state was measured by an infrared heat distribution test system. As shown in Fig. 3(c), the surface temperature of the UVC-LED ILS rose rapidly when it was turned on, then reached its maximum after about 10 min. It is worth noting that the temperature distribution of the UVC-LED ILS in working state is not uniform. Obviously, the temperature in the center of light-emitting area (p1) is higher than that of any other parts, but it is still less than 90 °C (acceptable for continuous work), which could be attributed to the excellent heat dissipation capability of the underneath AlN ceramic substrate and cooling system. In addition, the temperature of AlN ceramic substrate close to the light-emitting area (p3) is slightly higher than that of the edge part of quartz glass (p2) which is on the light-emitting area, further demonstrating the high thermal conductivity of AlN ceramic substrate. For other two UVC-LED ILSs, it’ reasonable to infer that the irradiance and temperature features would display a similar tendency. Overall, our results demonstrate that it’s essential to employ AlN ceramic with high thermal conductivity as the substrate to eliminate the temperature rise of UVC-LED ILSs. Other ideas such as more suitable chip arrangement would also do favor to the heat dissipation of UVC-LED ILSs, which will not be discussed further here.
Surface and air sterilization test
After the above characterizations of three UVC-LED ILSs, sterilization tests of them on Staphylococcus aureus were conducted subsequently to compare the sterilization effect of UVC light with different wavelengths. For all three experimental groups, the distance between the tested bacterial colony and the UVC-LED ILSs was set to 20 cm. By adjusting the injection current of three UVC-LED ILSs, the UVC irradiance on the bacterial colony surface was maintained at the same value of 0.50 mW/cm2. The sterilization time was also set to the same (10 s) to make sure that the bacterial colonies accept the same dose of UVC radiation. As shown in Table 1, with the same radiation dose, the inactivation rates of Staphylococcus aureus for 275, 265 and 255 nm UVC light were 96.71%, 99.57% and 99.76%, respectively. The result demonstrates that microorganisms, specifically, bacteria may be more sensitive to UV light around 260 nm, which is consistent with the absorption peak of DNA according to previous reports[33, 34].
Test microorganism | Test group | Test time (min) | Control
(w/o irradiation)
| | Air sterilization module |
---|
Colony count (CFU/m3)
| Nature inactivation rate (%) | | Colony count (CFU/m3)
| Inactivation rate (%) |
---|
Staphylococcus albus | 1 | 0 | 1.26 × 105 | 17.46 | | 1.18 × 105 | 97.03 |
30 | 1.04 × 105 | 2.89 × 103 |
2 | 0 | 1.18 × 105 | 17.88 | | 1.29 × 105 | 97.19 |
30 | 0.97 × 105 | | 2.98 × 103 |
3 | 0 | 1.23 × 105 | 17.89 | | 1.30 × 105 | 97.14 |
30 | 1.01 × 105 | | 3.05 × 103 |
Table 0. Sterilization effects of the air sterilization module based on the 275-nm-UVC-LED ILS on Staphylococcus albus in the air of 20 m3 confined room.
Although 275 nm may not be the most effective sterilization wavelength, 275 nm UVC-LEDs are still the mainstream product in current market for sterilization application. Therefore, we focused on the 275-nm-UVC-LED ILS and further tested its sterilization ability on different microorganisms. Escherichia coli as the representative of intestinal bacteria, Staphylococcus aureus as the representative of pyogenes and Candida albicans as the representative of pathogenic fungi were selected as the test microorganisms. The injection current of the 275-nm-UVC-LED ILS was set to 0.8 A, and the sterilization distance was set to 5 cm. In this case, the UVC irradiance on the microorganisms was estimated to be 18.5 mW/cm2 according to the data in Fig. 3(d). As can be seen in Fig. 4 and Table 2, the 275-nm-UVC-LED ILS achieved over 99.99% inactivation rate against Escherichia coli and Staphylococcus aureus and 99.90% inactivation rate against Candida albicans within only 1 s, exhibiting its efficient and rapid sterilization and disinfection capabilities. It’s worth noting that larger UVC radiation dose is required to achieve the same inactivation rate for Candida albicans, which may be due to the truth that fungi have cell walls.
Test microorganism | Test group | Test time (min) | Control
(w/o irradiation)
| | Air sterilization module |
---|
Colony count (CFU/m3)
| Nature inactivation rate (%) | | Colony count (CFU/m3)
| Inactivation rate (%) |
---|
Staphylococcus albus | 1 | 0 | 1.26 × 105 | 17.46 | | 1.18 × 105 | 97.03 |
30 | 1.04 × 105 | 2.89 × 103 |
2 | 0 | 1.18 × 105 | 17.88 | | 1.29 × 105 | 97.19 |
30 | 0.97 × 105 | | 2.98 × 103 |
3 | 0 | 1.23 × 105 | 17.89 | | 1.30 × 105 | 97.14 |
30 | 1.01 × 105 | | 3.05 × 103 |
Table 0. Sterilization effects of the air sterilization module based on the 275-nm-UVC-LED ILS on Staphylococcus albus in the air of 20 m3 confined room.
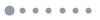
Figure 4.Micrographs of the Microorganism Colonies of (a, d) Escherichia coli, (b, e) Staphylococcus aureus, (c, f) Candida albicans before (a, b, c) and after (d, e, f) one-second sterilization by the 275-nm-UVC-LED ILS.
Based on the excellent surface sterilization ability of the 275-nm-UVC-LED ILS, we tried to promote it to air sterilization applications. An air sterilization module based on the 275-nm-UVC-LED ILS was well designed by combining with an L-shape air tube. The schematic diagram is presented in Fig. 5(a). Firstly, the contaminated air is continuously blown into the air tube by a fan installed at the inlet. Then, the contaminated air will be sterilized when it passes through the high-intensity ultraviolet irradiation area formed by the UVC-LED ILS installed at the corner. Finally, the purified air will be released out from the outlet. Fig. 5(b) shows a photo of the assembled air sterilization module. The air tube was made of polished Al with a high reflectivity to UVC light, which is helpful to get higher ultraviolet irradiance in the air tube. One can see several images of the UVC-LED array reflected by the polished Al tube wall from the inlet direction, as shown in Fig. 5(c). Meanwhile, the air tube was designed to L-shape to make sure that the contaminated air will flow along the normal direction of light-emitting surface of the UVC-LED ILS. In this case, the contaminated air could receive the maximum dose of ultraviolet irradiation, therefore achieving the best sterilization effect. By employing Staphylococcus albus, a major cause of nosocomial infections, as the test microorganism, air sterilization tests of this air sterilization module were carried out and repeated for three times to get a more accurate and reliable result. As shown in Table 3, an average inactivation rate of 97.12% against Staphylococcus albus in the air of 20 m3 confined room was achieved within 30 min treatment. It’s feasible to further increase the air sterilization efficiency by increasing the LOP of the UVC-LED ILS and designing different shapes or sizes of the air tube. Our air sterilization module based on UVC-LED ILS shows a great application potential in sterilization of confined spaces such as carriages and airplanes.
Test microorganism | Test group | Test time (min) | Control
(w/o irradiation)
| | Air sterilization module |
---|
Colony count (CFU/m3)
| Nature inactivation rate (%) | | Colony count (CFU/m3)
| Inactivation rate (%) |
---|
Staphylococcus albus | 1 | 0 | 1.26 × 105 | 17.46 | | 1.18 × 105 | 97.03 |
30 | 1.04 × 105 | 2.89 × 103 |
2 | 0 | 1.18 × 105 | 17.88 | | 1.29 × 105 | 97.19 |
30 | 0.97 × 105 | | 2.98 × 103 |
3 | 0 | 1.23 × 105 | 17.89 | | 1.30 × 105 | 97.14 |
30 | 1.01 × 105 | | 3.05 × 103 |
Table 0. Sterilization effects of the air sterilization module based on the 275-nm-UVC-LED ILS on Staphylococcus albus in the air of 20 m3 confined room.
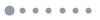
Figure 5.(Color online) (a) Schematic diagram and (b) photo of the air sterilization module based on 275-nm-UVC-LED ILS. (c) Photo of UVC-LED array viewed from the inlet direction.
Conclusion
In summary, three watt-level UVC-LED ILSs with different wavelengths were fabricated for efficient sterilization. By integrating 195 UVC-LED chips into one light source, a high LOP of 1.88 W was achieved under an injection current of 1.3 A. The UVC-LED ILSs show great irradiation uniformity and heat dissipation. What’s more, the UVC-LED ILSs were verified to have excellent sterilization capability. For surface sterilization, over 99.9% inactivation rate of several pathogenic microorganisms was achieved in only 1 s. For air sterilization, by combing with a well-designed L-shape air tube, the UVC-LED ILS based air sterilization module achieve more than 97% inactivation of Staphylococcus albus in air of 20 m3 confined space within 30 min. Our work demonstrates the importance of high integration for UVC-LEDs in sterilization, pointing out a way for UVC-LEDs in future sterilization applications.
Acknowledgements
This work was supported by the Guangdong Basic and Application Basic Research Foundation of Guangdong Province (Nos. 2021A1515111149, 2021B1515120022, 2020B010174003).
References
[1] R Abbasi, R Jain, K Nelson et al. Polymeric films containing sodium chlorite that release disinfectant gas upon activation with UV light. Adv Funct Mater, 29, 1804851(2019).
[2] N Ogata, M Sakasegawa, T Miura et al. Inactivation of airborne bacteria and viruses using extremely low concentrations of chlorine dioxide gas. Pharmacology, 97, 301(2016).
[3] A M Rauth. The physical state of viral nucleic acid and the sensitivity of viruses to ultraviolet light. Biophys J, 5, 257(1965).
[4] S E Beck, H B Wright, T M Hargy et al. Action spectra for validation of pathogen disinfection in medium-pressure ultraviolet (UV) systems. Water Res, 70, 27(2015).
[5] A L Santos, C Moreirinha, D Lopes et al. Effects of UV radiation on the lipids and proteins of bacteria studied by mid-infrared spectroscopy. Environ Sci Technol, 47, 6306(2013).
[6] W Hijnen, E Beerendonk, G J Medema. Inactivation credit of UV radiation for viruses, bacteria and protozoan (oo) cysts in water: a review. Water Res, 40, 3(2006).
[7] D Sarigiannis, S Karakitsios, M Antonakopoulou et al. Exposure analysis of accidental release of mercury from compact fluorescent lamps (CFLs). Sci Total Environ, 435, 306(2012).
[8] S Schalk, V Adam, E Arnold et al. UV-lamps for disinfection and advanced oxidation-lamp types, technologies and applications. IUVA News, 8, 32(2006).
[9] J F da Silveira Petruci, P R Fortes, V Kokoric et al. Real-time monitoring of ozone in air using substrate-integrated hollow waveguide mid-infrared sensors. Sci Reports, 3, 1(2013).
[10] H Hirayama, N Maeda, S Fujikawa et al. Recent progress and future prospects of AlGaN-based high-efficiency deep-ultraviolet light-emitting diodes. Jpn J Appl Phys, 53, 100209(2014).
[11] D Li, K Jiang, X Sun et al. AlGaN photonics: recent advances in materials and ultraviolet devices. Adv Opt Photonics, 10, 43(2018).
[12] M Kneissl, T Y Seong, J Han et al. The emergence and prospects of deep-ultraviolet light-emitting diode technologies. Nat Photonics, 13, 233(2019).
[13] Y Taniyasu, M Kasu, T Makimoto. An aluminium nitride light-emitting diode with a wavelength of 210 nanometres. Nature, 441, 325(2006).
[14] A Khan, K Balakrishnan, T Katona. Ultraviolet light-emitting diodes based on group three nitrides. Nat Photonics, 2, 77(2008).
[15] Y Nagasawa, A Hirano. A review of AlGaN-based deep-ultraviolet light-emitting diodes on sapphire. Appl Sci, 8, 1264(2018).
[16] J Simon, V Protasenko, C Lian et al. Polarization-induced hole doping in wide–band-gap uniaxial semiconductor heterostructures. Science, 327, 60(2010).
[17] L Zhang, K Ding, J Yan et al. Three-dimensional hole gas induced by polarization in (0001)-oriented metal-face III-nitride structure. Appl Phys Lett, 97, 062103(2010).
[18] H Y Ryu, I G Choi, H S Choi et al. Investigation of light extraction efficiency in AlGaN deep-ultraviolet light-emitting diodes. Appl Phys Express, 6, 062101(2013).
[19] Y Li, C Wang, Y Zhang et al. Analysis of TM/TE mode enhancement and droop reduction by a nanoporous n-AlGaN underlayer in a 290 nm UV-LED. Photonics Res, 8, 806(2020).
[20] C Bowker, A Sain, M Shatalov et al. Microbial UV fluence-response assessment using a novel UV-LED collimated beam system. Water Res, 45, 2011(2011).
[21] J R Bolton, K G Linden. Standardization of methods for fluence (UV dose) determination in bench-scale UV experiments. J Environ Eng, 129, 209(2003).
[22] A Suzuki, A Emoto, A Shirai et al. Ultraviolet light-emitting diode (UV-LED) sterilization of citrus bacterial canker disease targeted for effective decontamination of citrus sudachi fruit. Biocontrol Sci, 27, 1(2022).
[23] Y W Lee, H D Yoon, J H Park et al. Application of 265-nm UVC LED lighting to sterilization of typical gram negative and positive bacteria. J Korean Physl Soc, 72, 1174(2018).
[24] S Y Huang, J C Lin, X Q Huang et al. Large-area 280 nm LED flexible sterilization light source with improved thermal performance. Optik, 248, 168109(2021).
[25] B S Kim, S Youm, Y K Kim. Sterilization of harmful microorganisms in hydroponic cultivation using an ultraviolet LED light source. Sens Mater, 32, 3773(2020).
[26] S S Nunayon, H Zhang, A C K Lai. Comparison of disinfection performance of UVC-LED and conventional upper-room UVGI systems. Indoor Air, 30, 180(2020).
[27] S Liu, W Luo, D Li et al. Sec-eliminating the SARS-CoV-2 by AlGaN based high power deep ultraviolet light source. Adv Funct Mater, 31, 2008452(2020).
[28] M Bormann, M Alt, L Schipper et al. Disinfection of SARS-CoV-2 contaminated surfaces of personal items with UVC-LED disinfection boxes. Viruses, 13, 598(2021).
[29] G Tamulaitis, J Mickevičius, K Kazlauskas et al. Efficiency droop in high-Al-content AlGaN/AlGaN quantum wells. Phys Status Solidi C, 8, 2130(2011).
[30] J Yun, J I Shim, H Hirayama. Analysis of efficiency droop in 280-nm AlGaN multiple-quantum-well light-emitting diodes based on carrier rate equation. Appl Phys Express, 8, 022104(2015).
[31] J Cho, E F Schubert, J K Kim. Efficiency droop in light-emitting diodes: Challenges and countermeasures. Laser Photonics Rev, 7, 408(2013).
[32] J Hu, J Zhang, Y Zhang et al. Enhanced performance of AlGaN-based deep ultraviolet light-emitting diodes with chirped superlattice electron deceleration layer. Nanoscale Res Lett, 14, 1(2019).
[33] J R Bolton, C A Cotton. The ultraviolet disinfection handbook. Glacier Publishing Services, Inc.(2011).
[34] R P Rastogi, A Kumar, M B Tyagi et al. Molecular mechanisms of ultraviolet radiation-induced DNA damage and repair. J Nucleic Acids, 90, 560(2010).