Anjin Liu, Philip Wolf, James A. Lott, Dieter Bimberg, "Vertical-cavity surface-emitting lasers for data communication and sensing," Photonics Res. 7, 121 (2019)

Search by keywords or author
- Photonics Research
- Vol. 7, Issue 2, 121 (2019)
![Schematic of a top-emitting VCSEL [19]. Inset is a scanning electron microscope image of the cross section of a high-speed VCSEL after it is cleaved.](/richHtml/prj/2019/7/2/02000121/img_001.jpg)
Fig. 1. Schematic of a top-emitting VCSEL [19]. Inset is a scanning electron microscope image of the cross section of a high-speed VCSEL after it is cleaved.
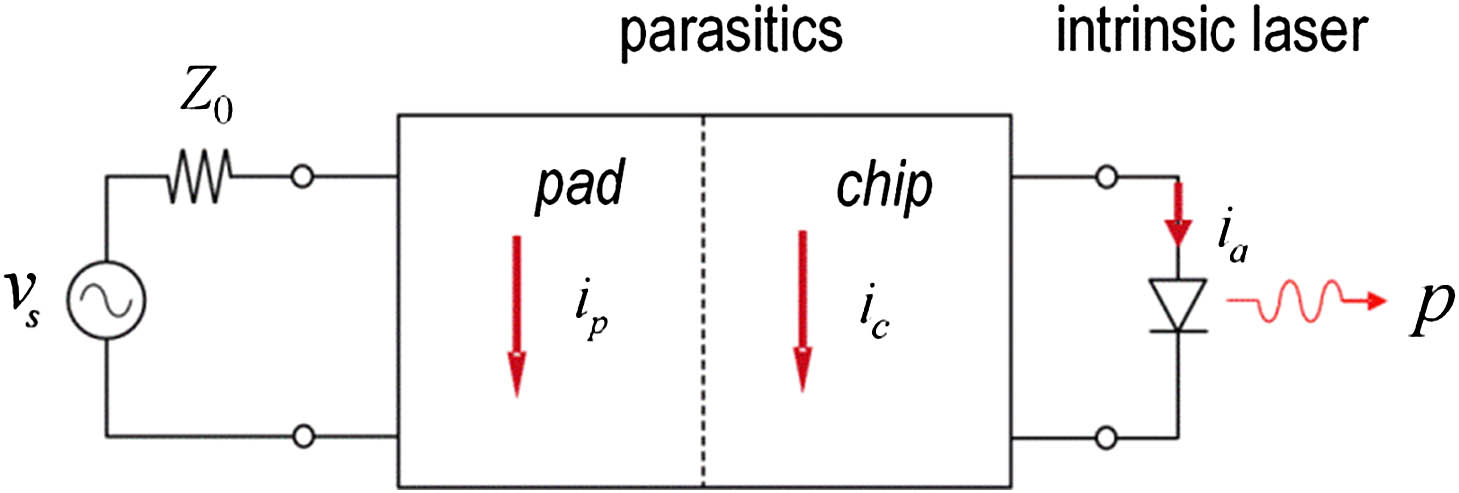
Fig. 2. Small-signal model of a VCSEL with the high-frequency driving source.
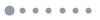
Fig. 3. Schematic of representative optical modes (straight lines) and gain spectra (curves) behavior in a VCSEL as functions of increasing temperature. T 0 denotes the typical room temperature.
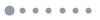
Fig. 4. Simulated PAM4 and on–off keying (OOK) eye diagrams at 40 Gbps with a constant modulation bandwidth of 20 GHz.
![(a) End-to-end coupling between a VCSEL and a PIC based on an SOI platform [115]. A spot-size convertor in the PIC side is always adopted for a high coupling efficiency between the VCSEL and the silicon waveguide. (b) VCSEL coupled to a PIC by 45° micro-reflectors [116]. (c) Grating coupler for coupling between a VCSEL and a PIC [123]. (d) Photonic wire bond for integration for a surface-emitting laser and a PIC [127]. The laser can be a VCSEL or a distributed-feedback surface-emitting laser. PWB, photonic wire bond.](/Images/icon/loading.gif)
Fig. 5. (a) End-to-end coupling between a VCSEL and a PIC based on an SOI platform [115]. A spot-size convertor in the PIC side is always adopted for a high coupling efficiency between the VCSEL and the silicon waveguide. (b) VCSEL coupled to a PIC by 45° micro-reflectors [116]. (c) Grating coupler for coupling between a VCSEL and a PIC [123]. (d) Photonic wire bond for integration for a surface-emitting laser and a PIC [127]. The laser can be a VCSEL or a distributed-feedback surface-emitting laser. PWB, photonic wire bond.
![Schematic of a tracking system based on SMI [129,130].](/Images/icon/loading.gif)
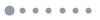
Fig. 7. Components of a face recognition module in a modern smartphone. (a) VCSELs for time-of-flight (ToF) proximity sensing and IR illumination. (b) VCSEL array for projection of randomly distributed dots to sense object distance information.
![(a) Schematic of focal plane scanning [148,151]. (b) Illustration of structured light [153].](/Images/icon/loading.gif)
Fig. 8. (a) Schematic of focal plane scanning [148,151]. (b) Illustration of structured light [153].
![(a) Schematic of an HCG. The red arrows show the direction of wave incidence. The black arrows indicate the E-field direction in both TE and TM polarizations of incidence. (b) Double-mode solution exhibiting perfect intensity cancellation at the HCG output plane leading to 100% reflectivity [159].](/Images/icon/loading.gif)
Fig. 9. (a) Schematic of an HCG. The red arrows show the direction of wave incidence. The black arrows indicate the E-field direction in both TE and TM polarizations of incidence. (b) Double-mode solution exhibiting perfect intensity cancellation at the HCG output plane leading to 100% reflectivity [159].
![(a) Schematic of an HCG-VCSEL [160]. (b) HCG-VCSEL array for single-lobe, double-lobe, triple-lobe, “bow-tie,” “sugar cone,” and “doughnut” beam patterns [177]. (c) Schematic of a nanoelectromechanical tunable VCSEL using the highly reflective HCG as its top mirror, instead of conventional DBRs [180]. (d) Schematic of a monolithic HCG-VCSEL array with different HCG parameters.](/Images/icon/loading.gif)
Fig. 10. (a) Schematic of an HCG-VCSEL [160]. (b) HCG-VCSEL array for single-lobe, double-lobe, triple-lobe, “bow-tie,” “sugar cone,” and “doughnut” beam patterns [177]. (c) Schematic of a nanoelectromechanical tunable VCSEL using the highly reflective HCG as its top mirror, instead of conventional DBRs [180]. (d) Schematic of a monolithic HCG-VCSEL array with different HCG parameters.
![(a) Schematic of a VCSEL with a silicon HCG as a bottom mirror. An HCG serves as the bottom mirror and potentially serves as a waveguide coupler for an in-plane SOI waveguide, facilitating the integration of a VCSEL with in-plane silicon photonic circuits [188]. (b) Schematic of a vertical-cavity laser with lateral emission into a silicon waveguide via an HCG [189]. (c) Schematic of a vertical-cavity laser with in-plane out-coupling into a SiN waveguide. A subwavelength grating is inserted under a half-VCSEL to redirect the vertical resonance light to the in-plane SiN waveguide [192].](/Images/icon/loading.gif)
Fig. 11. (a) Schematic of a VCSEL with a silicon HCG as a bottom mirror. An HCG serves as the bottom mirror and potentially serves as a waveguide coupler for an in-plane SOI waveguide, facilitating the integration of a VCSEL with in-plane silicon photonic circuits [188]. (b) Schematic of a vertical-cavity laser with lateral emission into a silicon waveguide via an HCG [189]. (c) Schematic of a vertical-cavity laser with in-plane out-coupling into a SiN waveguide. A subwavelength grating is inserted under a half-VCSEL to redirect the vertical resonance light to the in-plane SiN waveguide [192].
|
Table 1. Modulation Bandwidths and Bit Rates of VCSELs at Room Temperature Using the Standard On–Off Keying in a Back-to-Back Data Transmission Configuration
|
Table 2. Selected Results on Bandwidths and Bit Rates of VCSELs at High Temperatures in an On–Off Keying Modulation Format for Back-to-Back Data Transmission Configuration
|
Table 3. Energy Efficiencies of High-Speed VCSELs with the On–Off Keying Modulation Format in a Back-to-Back Data Transmission Configuration
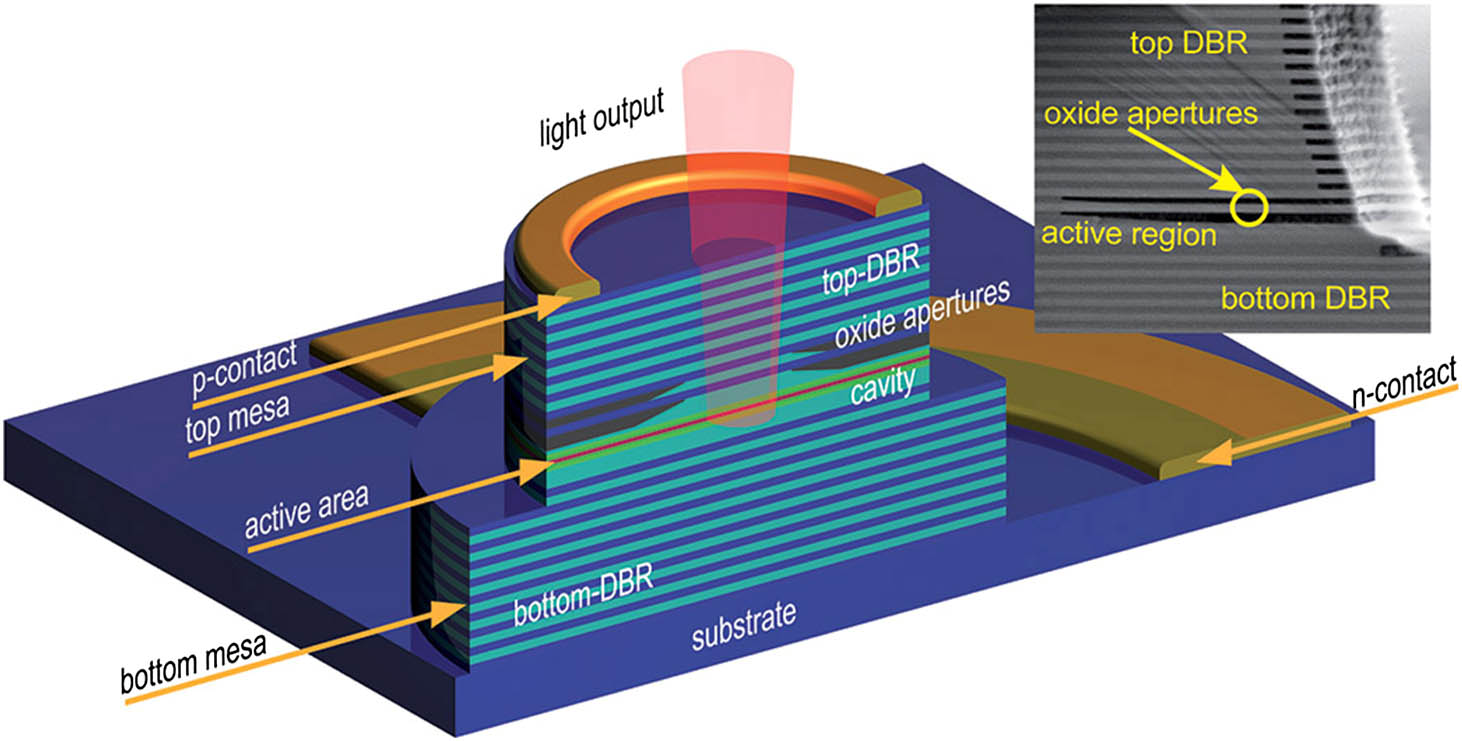
Set citation alerts for the article
Please enter your email address