
- Photonics Research
- Vol. 7, Issue 2, 121 (2019)
Abstract
1. INTRODUCTION
Semiconductor heterostructure lasers are key enabling devices in our Internet society governed by the science of light: photonics. Outstanding properties of such lasers are the high efficiency of the conversion of electrical energy to photons, the small footprint, the modulation bandwidth, the excellent reliability, and the low cost. Important applications include optical communication, optical storage, sensing, printing, pumping for other lasers, and micro-invasive surgery. The vertical-cavity surface-emitting laser (VCSEL) arose from the ideas and contributions of several research groups. The first semiconductor laser emitting perpendicular to the surface of a semiconductor wafer traces back to the work of Melngailis in Ref. [
A modern VCSEL is constructed by two DBRs aligned in the vertical direction to form a vertical laser cavity, and the light output is along the same vertical wafer epitaxial growth direction normal to the wafer. Such a unique device structure is very different from an edge-emitting laser, which endows a VCSEL with many notable advantages and performance attributes as compared to other types of laser diodes. The small cavity volume brings a low threshold current, high quantum efficiency, and high-speed modulation at a low current. The symmetric transverse structure and surface emission of a VCSEL lead to a circular and non-astigmatic beam, good for coupling to an optical fiber and other optics. Surface emission also enables a large-scale two-dimensional (2D) VCSEL array and wafer level fabrication and testing for an overall low cost of manufacturing.
VCSELs have experienced exponential growth in key photonics application areas, for example, in data communication, optical sensing, laser printing, light detection and ranging (LiDAR), and illumination, to mention a few [
Sign up for Photonics Research TOC. Get the latest issue of Photonics Research delivered right to you!Sign up now
2. FUNDAMENTALS OF VCSELs
A. Structure of VCSELs
The modern VCSEL is constructed by combining a gain region within an optical cavity with two highly reflective DBRs to form a vertical resonator, as shown in Fig.
Figure 1.Schematic of a top-emitting VCSEL [19]. Inset is a scanning electron microscope image of the cross section of a high-speed VCSEL after it is cleaved.
B. Dynamics of VCSELs
Figure
Figure 2.Small-signal model of a VCSEL with the high-frequency driving source.
The relaxation resonance frequency is an oscillation frequency between the carriers and photons that interact via stimulated emission in the laser cavity. The relaxation resonance frequency increases with the square root of the bias current and is formulated as
The damping factor
To achieve a high modulation bandwidth, a large
Electrical parasitics including bonding pads eventually limit the modulation bandwidth of a VCSEL. The transfer function introduced by electrical parasitics can be expressed by a single-pole low-pass filter transfer function as
The overall electrical small-signal modulation response of a VCSEL is given by multiplying the intrinsic transfer function with the transfer function introduced by the electrical parasitics, and thus
3. VCSELs FOR DATA COMMUNICATION
VCSELs are the dominant optical sources for multimode fiber (MMF)-based optical links in data centers and high-performance computers (HPCs). Since the network traffic exponentially increases year by year, wide modulation bandwidth is an essential requirement to meet the thirst for ever higher transmission data rates, and more and more power in data centers and HPCs is consumed. The heat dissipated leads to an operating environment reaching temperatures of 85°C, even with advanced cooling technologies. The latest communication system designs seek to develop VCSELs that are more energy efficient and that are capable of maintaining their room temperature modulation bit rates at high temperatures without adjusting the operating parameters.
A. Modulation Speeds of VCSELs
Table
Group | Bandwidth (GHz) | Bit Rate (Gbps) | Temperature (°C) | Oxide Aperture (μm) | Year | Refs. | |
IBM | 850 | 15.4 | 20 | 25 | 8 | 2001 | [ |
Finisar-IBM | 850 | 19 | 30 | 25 | 6 | 2008 | [ |
CUT | 850 | 20 | 32 | 25 | 9 | 2009 | [ |
CUT | 850 | 23 | 40 | 25 | 7 | 2010 | [ |
CUT | 850 | 28 | 44 | 25 | 7 | 2012 | [ |
CUT | 850 | 24 | 57 | 25 | 8 | 2013 | [ |
CUT | 850 | 30 | 50 | 25 | 3.5 | 2015 | [ |
TU Berlin | 850 | 20 | 30 | 25 | 6 | 2009 | [ |
TU Berlin | 850 | 40 | 25 | 9 | 2009 | [ | |
UIUC | 850 | 21.2 | 40 | 20 | 4 | 2014 | [ |
UIUC | 850 | 29.2 | 57 | 25 | 5 | 2016 | [ |
NCU | 850 | 22.4 | 40 | 25 | 4 | 2013 | [ |
NCU | 850 | 26 | 41 | 25 | 8 | 2015 | [ |
UCSB | 980 | >20 | 35 | 20 | 3 | 2007 | [ |
TU Berlin | 980 | 44 | 25 | 6 | 2011 | [ | |
TU Berlin | 980 | 24.7 | 50 | 25 | 5 | 2014 | [ |
TU Berlin | 980 | 26.6 | 52 | 25 | 6 | 2016 | [ |
TU Berlin | 980 | 35.5 | 25 | 3 | 2018 | [ | |
CUT | 1060 | 22 | 50 | 25 | 4 | 2017 | [ |
NEC | 1100 | 20 | 25 | 25 | 6.9 | 2006 | [ |
NEC | 1100 | 24 | 30 | 25 | 6 | 2007 | [ |
NEC | 1100 | 24 | 40 | 25 | 6 | 2008 | [ |
Table 1. Modulation Bandwidths and Bit Rates of VCSELs at Room Temperature Using the Standard On–Off Keying in a Back-to-Back Data Transmission Configuration
Other groups also reported many exciting results on high-speed VCSELs in the 850 nm range. IBM and Finisar jointly reported a 55 Gbps directly modulated 850 nm VCSEL-based optical link in 2012 and a 56.1 Gbps link in 2013 [
VCSELs emitting at or in the range 980 to 1100 nm are now becoming important for data communication, most especially for wavelength division multiplexing and free-space optical communication [
In 2007, error-free operation at 35 Gbps was demonstrated with a 980 nm VCSEL by the Coldren group at the University of California Santa Barbara (UCSB). This VCSEL used a tapered oxide aperture to reduce optical loss, multiple deep oxide layers to reduce the parasitic capacitance, and an optimized p-doping profile in the top DBRs to lower the loss and the resistance, showing a 3 dB modulation bandwidth larger than 20 GHz [
For VCSELs emitting at or near 1100 nm, Nippon Electric Company Ltd. (NEC) demonstrated error-free 25 Gbps operation at 25°C and a 3 dB modulation bandwidth up to 20 GHz in 2006 [
Currently, over 90% of the MMF links are less than 100 m [
Efforts have also been carried out to explore unconventional VCSELs with transverse coupled cavities for additional photon–photon resonances to extend the modulation bandwidth [
B. High-Temperature VCSELs
The ambient temperature can reach 85°C in data centers and HPCs, even with advanced cooling technology. Such a temperature might deteriorate the performance (data rate, energy consumption, reliability). VCSELs can maintain the room temperature performance without adjusting driving conditions and adding extra cooling systems by detuning the laser cavity resonance from the gain peak [
Figure 3.Schematic of representative optical modes (straight lines) and gain spectra (curves) behavior in a VCSEL as functions of increasing temperature.
Group | Bandwidth (GHz) | Bit Rate (Gbps) | Temperature (°C) | Oxide Aperture (μm) | Year | Refs. | |
Finisar | 850 | 10 | 14 | 95 | 8 | 2012 | [ |
Emcore | 850 | 16 | 28 | 85 | 7.5 | 2013 | [ |
CUT | 850 | 21 | 40 | 85 | 7 | 2013 | [ |
IBM-CUT | 850 | 21 | 50 | 90 | 6 | 2015 | [ |
UIUC | 850 | 24.5 | 50 | 85 | 5 | 2016 | [ |
NCU | 850 | 22.4 | 34 | 85 | 4 | 2013 | [ |
NCU | 850 | 20 | 41 | 85 | 8 | 2015 | [ |
VIS | 850 | 25 | 150 | 4 | 2018 | [ | |
VIS | 850 | 25 | 130 | 4 | 2018 | [ | |
TU Berlin | 980 | 11 | 20 | 120 | 3 | 2008 | [ |
TU Berlin | 980 | 38 | 85 | 6 | 2011 | [ | |
TU Berlin | 980 | 30 | 120 | 6 | 2011 | [ | |
TU Berlin | 980 | 23 | 46 | 85 | 5 | 2014 | [ |
TU Berlin | 980 | 38 | 85 | 5.5 | 2014 | [ | |
TU Berlin | 980 | 18 | 35 | 85 | 3 | 2014 | [ |
TU Berlin | 980 | 24.5 | 50 | 85 | 6 | 2016 | [ |
CUT | 1060 | 16 | 40 | 85 | 4 | 2017 | [ |
Table 2. Selected Results on Bandwidths and Bit Rates of VCSELs at High Temperatures in an On–Off Keying Modulation Format for Back-to-Back Data Transmission Configuration
C. Energy Efficiency of VCSELs
Power consumption becomes more and more important for both data centers and HPCs. Large power consumption increases the economic and ecological cost. Large power consumption might also deteriorate the long-term stability of VCSELs. The energy-to-data rate ratio
Large energy efficiency was reported for small oxide aperture diameter VCSELs [
Group | Bit Rate (Gbps) | Temperature (°C) | Energy eff. (fJ/bit) | Oxide Aperture (μm) | Year | Refs. | |
TU Berlin-VIS | 850 | 25 | 25 | 99 | 2 | 2011 | [ |
TU Berlin-VIS | 850 | 17 | 25 | 69 | 2 | 2011 | [ |
TU Berlin-VIS | 850 | 25 | 25 | 56 | 3.5 | 2012 | [ |
TU Berlin | 850 | 40 | 25 | 108 | 4 | 2013 | [ |
CUT | 850 | 50 | 25 | 95 | 3.5 | 2015 | [ |
CUT | 850 | 40 | 25 | 73 | 3.5 | 2015 | [ |
UIUC | 850 | 40 | 20 | 395 | 4 | 2014 | [ |
NCU | 850 | 12.5 | 25 | 109 | 6 | 2011 | [ |
NCU | 850 | 34 | 25 | 345 | 6 | 2011 | [ |
NCU | 850 | 34 | 25 | 107 | 4 | 2013 | [ |
UCSB | 980 | 35 | 20 | 286 | 3 | 2009 | [ |
TU Berlin | 980 | 38 | 85 | 177 | 5.5 | 2014 | [ |
TU Berlin | 980 | 35 | 85 | 139 | 3 | 2014 | [ |
TU Berlin | 980 | 35 | 25 | 145 | 3 | 2015 | [ |
Furukawa | 1060 | 10 | 25 | 140 | 2011 | [ | |
Furukawa | 1060 | 25 | 25 | 76 | 2014 | [ | |
CUT | 1060 | 50 | 25 | 100 | 4 | 2017 | [ |
Table 3. Energy Efficiencies of High-Speed VCSELs with the On–Off Keying Modulation Format in a Back-to-Back Data Transmission Configuration
D. VCSELs with PAM4 Modulation Format
Today, short-reach optical interconnects are dominated by VCSELs combined with MMF and direct detection, offering low cost and small form factor. Existing IEEE standards (802.3bm) support 10 Gbps and 25 Gbps core rates in 4-fiber and 10-fiber arrangements to support up to 100 Gbps of traffic. However, a 400 GbE (Gigabit Ethernet supporting 400 Gbps) solution as envisioned by the IEEE Task Force (P802.3bs) based on 25 Gbps will require 16 fibers in each direction. Alternative solutions that lead to reduced fiber numbers could include higher bit rates combined with higher modulation formats to end up at 50+ Gbps per line. Four-level pulse amplitude modulation (PAM4) [
Combining PAM4 and digital signal processing (DSP) techniques with coarse wavelength division multiplexing (CWDM, O-band) or short wavelength division multiplexing (SWDM) is enabling the next generation of high-speed optical VCSEL-based interconnects. Compared to the on–off keying modulation format, PAM is a way to transmit more bits into the same amount of time on a serial channel by using different signaling levels, as shown in Fig.
Figure 4.Simulated PAM4 and on–off keying (OOK) eye diagrams at 40 Gbps with a constant modulation bandwidth of 20 GHz.
Recently, up to 94 Gbps PAM4 was demonstrated over single wavelengths using 850 nm VCSELs with a 20 GHz 3 dB bandwidth using energy expensive pre-emphasis and receiver equalization [
E. VCSELs for Photonic Integrated Circuits
VCSELs are ideal sources for photonic integrated circuits (PICs). However, a VCSEL emits perpendicular to the wafer plane, while a PIC lies in the wafer plane. There are at least four approaches for coupling light from VCSELs to an in-plane PIC, as shown in Fig.
Figure 5.(a) End-to-end coupling between a VCSEL and a PIC based on an SOI platform [115]. A spot-size convertor in the PIC side is always adopted for a high coupling efficiency between the VCSEL and the silicon waveguide. (b) VCSEL coupled to a PIC by 45° micro-reflectors [116]. (c) Grating coupler for coupling between a VCSEL and a PIC [123]. (d) Photonic wire bond for integration for a surface-emitting laser and a PIC [127]. The laser can be a VCSEL or a distributed-feedback surface-emitting laser. PWB, photonic wire bond.
4. VCSELs FOR SENSING
VCSELs show superior beam quality, low energy consumption, low cost, high reliability, and can be easily integrated to form 2D arrays, all requirements for sensing applications like mice or smartphones.
A. VCSEL-Based Sensors with Self-Mixing Interferometry
VCSELs used in optical mice first appeared in 2000 [
Figure 6.Schematic of a tracking system based on SMI [129,130].
VCSEL-based sensors based on SMI have many industrial applications in, e.g., cable manufacturing; extrusion; steel, paper processing and other production equipment; and in measuring length and movements of objects [
B. VCSEL-Based Sensors for Three-Dimensional Sensing
VCSELs have considerable potential in three-dimensional (3D) sensing, such as in gesture recognition, face recognition, eye movement tracking, as well as in 3D photography and autofocusing [
For 3D sensing applications, VCSELs have huge advantages as compared to competing approaches. VCSELs emit with a narrow spectral width of less than 1 nm, which is much lower than the emission width of LEDs, and have a temperature dependence of typically 0.06 nm/K [
There are primarily two types of methods for 3D sensing. The first one is the time-of-flight (ToF) method [
Figure 7.Components of a face recognition module in a modern smartphone. (a) VCSELs for time-of-flight (ToF) proximity sensing and IR illumination. (b) VCSEL array for projection of randomly distributed dots to sense object distance information.
The first ToF technology for 3D sensing is called a scanning ToF sensor, where single pixels are scanned one at a time with a VCSEL via short pulses. In a scanning ToF sensor, the pulse duration is always 1–10 ns, and the duty cycle is less than 10% [
Figure 8.(a) Schematic of focal plane scanning [148,151]. (b) Illustration of structured light [153].
An alternative method for 3D sensing uses structured light [
5. VCSELs WITH HIGH-CONTRAST METASTRUCTURES
Extensive research on surface metastructures or nanostructures has been carried out in the past decade, and many applications have been suggested to use their unique optical properties. High-index-contrast 1D wavelength gratings, also called high-contrast gratings (HCGs), and the 2D variation of such structures, generalized high-contrast metastructures (HCMs), have been and continue to be the focus of intense research. Here we focus on 1D HCGs and VCSELs with 1D HCGs. 2D HCMs have been comprehensively reviewed recently elsewhere [
A. Fundamentals of HCGs
A HCG reflector, also called a photonic crystal mirror or a guided-mode resonant reflector [
Figure 9.(a) Schematic of an HCG. The red arrows show the direction of wave incidence. The black arrows indicate the E-field direction in both TE and TM polarizations of incidence. (b) Double-mode solution exhibiting perfect intensity cancellation at the HCG output plane leading to 100% reflectivity [159].
For the HCG design, the structure parameters can be optimized by rigorous coupled wave analysis (RCWA) [
HCGs also show a strong ability to confine the field. The energy penetration depth of HCGs is much smaller than that of DBRs [
In fabricating HCG-VCSELs, a sacrificial layer below the HCG layer is always removed to create a suspended HCG surrounded by a low refractive index material (for example, air). Alternatively, epitaxial AlGaAs that is selectively oxidized to form the low refractive index material AlGaO may be used as the underlying low refractive index layer. Therefore, a lattice matched material system is essential from the perspective of both device performance and fabrication feasibility. Recently zero-contrast gratings (ZCGs) have been proposed to replace completely or partly the top DBR [
B. VCSELs with Metastructures
The first electrical HCG-VCSEL reported in 2007 was realized at 850 nm in the GaAs material system as shown in Fig.
Figure 10.(a) Schematic of an HCG-VCSEL [160]. (b) HCG-VCSEL array for single-lobe, double-lobe, triple-lobe, “bow-tie,” “sugar cone,” and “doughnut” beam patterns [177]. (c) Schematic of a nanoelectromechanical tunable VCSEL using the highly reflective HCG as its top mirror, instead of conventional DBRs [180]. (d) Schematic of a monolithic HCG-VCSEL array with different HCG parameters.
HCG reflectors are resonant structures, very different from DBRs, and the reflection phase can be tuned while keeping a high reflectivity [
Vertical coupling was also proposed to extract light from a Fabry–Perot microcavity composed of double HCG mirrors to a Si waveguide [
Figure 11.(a) Schematic of a VCSEL with a silicon HCG as a bottom mirror. An HCG serves as the bottom mirror and potentially serves as a waveguide coupler for an in-plane SOI waveguide, facilitating the integration of a VCSEL with in-plane silicon photonic circuits [188]. (b) Schematic of a vertical-cavity laser with lateral emission into a silicon waveguide via an HCG [189]. (c) Schematic of a vertical-cavity laser with in-plane out-coupling into a SiN waveguide. A subwavelength grating is inserted under a half-VCSEL to redirect the vertical resonance light to the in-plane SiN waveguide [192].
6. CONCLUSIONS AND PROSPECTS
VCSELs have unique advantages compared to edge-emitting lasers. Impressive progress has been achieved enabling energy-efficient and fast data communication in the past decade. Novel device designs based on the deeply developed insights to the device physics and to advanced modulation formats have been used to boost the performance of VCSELs. Data rates beyond 50 Gbps in the on–off keying modulation format have been demonstrated. An energy efficiency of close to 50 fJ/bit was reported at 25°C for a small oxide aperture VCSEL. An error-free transmission rate of 50 Gbps at 90°C was also demonstrated. To increase the capacity of MMF-based links, the PAM4 modulation format is employed, yielding a 100 Gbps data rate in a single lane. 400 Gbps via SWDM was also achieved.
VCSELs will continue to be the dominant laser sources in data centers and HPCs and might penetrate chip-to-chip and even on-chip optical interconnects. Single-mode VCSELs with a reduced spectral width will attract significant interest for extended distance transmission over MMF for data centers and HPCs [
VCSELs have unique advantages over silicon photonics technology, which is presently a hot topic [
VCSEL-based sensors have wide applications in consumer electronics, car markets, Internet of Things, atomic sensing [
References
[1] I. Melngailis. Longitudinal injection plasma laser of InSb. Appl. Phys. Lett., 6, 59-60(1965).
[18] F. Koyama. Recent advances of VCSEL photonics. J. Lightwave Technol., 24, 4502-4513(2006).
[19] H. Li, P. Wolf, P. Moser, G. Larisch, J. A. Lott, D. Bimberg. Vertical-cavity surface-emitting lasers for optical interconnects. SPIE Newsroom(2014).
[21] R. Michalzik. VCSELs - Fundamentals, Technology and Applications of Vertical-Cavity Surface-Emitting Lasers, 166(2013).
[22] J. A. Tatum. VCSEL proliferation. Proc. SPIE, 6484, 648403(2014).
[24] C. Wilmsen, H. Temkin, L. A. Coldren. Vertical Cavity Surface Emitting Lasers: Design, Fabrication, Characterization and Applications(1999).
[25] H. E. Li, K. Iga. Vertical-Cavity Surface-Emitting Laser Devices, 6(2003).
[34] L. A. Coldren, S. W. Corzine, M. L. Mašanović. Diode Lasers and Photonic Integrated Circuits(2012).
[40] W. Hofmann, P. Moser, P. Wolf, A. Mutig, M. Kroh, D. Bimberg. 44 Gb/s VCSEL for optical interconnects. Optical Fiber Communication Conference, PDPC5(2011).
[45] K. Lascola, W. Yuen, C. Chang-Hasnain. Structural dependence of the thermal resistance of vertical cavity surface emitting lasers. IEEE/LEOS Summer Topical Meeting, 79-80(1997).
[51] M. Azuchi, N. Jikutani, M. Ami, T. Kondo, F. Koyama. Multioxide layer vertical-cavity surface-emitting lasers with improved modulation bandwidth. 5th Pacific Rim Conference on Lasers and Electro-Optics, 1, 163(2003).
[54] D. M. Kuchta, P. Pepeljugoski, Y. Kwark. VCSEL modulation at 20 Gb/s over 200 m of multimode fiber using a 3.3 V SiGe laser driver IC. Digest of LEOS Summer Topical Meetings: Advanced Semiconductor Lasers and Applications/Ultraviolet and Blue Lasers and Their Applications/Ultralong Haul DWDM Transmission and Networking/WDM Compo, 49-50(2001).
[55] R. H. Johnson, D. M. Kuchta. 30 Gb/s directly modulated 850 nm datacom VCSELs. Conference on Lasers and Electro-Optics, CPDB2(2008).
[64] M. Liu, C. Y. Wang, M. Feng, N. Holonyak. 850 nm oxide-confined VCSELs with 50 Gb/s error-free transmission operating up to 85°C. Conference on Lasers and Electro-Optics, SF1L.6(2016).
[69] N. Haghighi, G. Larisch, R. Rosales, M. Zorn, J. A. Lott. 35 GHz bandwidth with directly current modulated 980 nm oxide aperture single cavity VCSELs. IEEE International Semiconductor Laser Conference (ISLC), WD4(2018).
[72] T. Anan, N. Suzuki, K. Yashiki, K. Fukatsu, H. Hatakeyama, T. Akagawa, K. Tokutome, M. Tsuji. High-speed 1.1-μm-range InGaAs VCSELs. Optical Fiber Communication Conference, OthS5(2008).
[75] D. M. Kuchta, A. V. Rylyakov, C. L. Schow, J. E. Proesel, C. Baks, C. Kocot, L. Graham, R. Johnson, G. Landry, E. Shaw, A. MacInnes, J. Tatum. A 55 Gb/s directly modulated 850 nm VCSEL-based optical link. IEEE Photonics Conference, PD1.5(2012).
[76] D. M. Kuchta, C. L. Schow, A. V. Rylyakov, J. E. Proesel, F. E. Doany, C. Baks, B. H. Hamel-Bissell, C. Kocot, L. Graham, R. Johnson, G. Landry, E. Shaw, A. MacInnes, J. Tatum. A 56.1 Gb/s NRZ modulated 850 nm VCSEL-based optical link. Optical Fiber Communication Conference, OW1B.5(2013).
[77] M. Liu, C. Y. Wang, M. Feng, N. Holonyak. 50 Gb/s error-free data transmission of 850 nm oxide-confined VCSELs. Optical Fiber Communication Conference, Tu3D.2(2016).
[83] N. Suzuki, H. Hatakeyama, K. Fukatsu, T. Anan, K. Yashiki, M. Tsuji. 25-Gbps operation of 1.1-μm-range InGaAs VCSELs for high-speed optical interconnections. Optical Fiber Communication Conference, OFA4(2006).
[85] H. Liu, C. F. Lam, C. Johnson. Scaling optical interconnects in datacenter networks opportunities and challenges for WDM. IEEE Symposium on High Performance Interconnects, 113-116(2010).
[105] J.-W. Shi, W.-C. Weng, F.-M. Kuo, J.-I. Chyi, S. Pinches, M. Geen, A. Joel. Oxide-relief vertical-cavity surface-emitting lasers with extremely high data-rate/power-dissipation ratios. Optical Fiber Communication Conference, OthG2(2011).
[109] J. Lavrencik, S. Varughese, V. A. Thomas, G. Landry, Y. Sun, R. Shubochkin, K. Balemarthy, J. Tatum, S. E. Ralph. 100 Gbps PAM-4 transmission over 100 m OM4 and wideband fiber using 850 nm VCSELs. European Conference and Exhibition on Optical Communication (ECOC), Th.1.C5(2016).
[110] J. Lavrencik, S. Varughese, V. A. Thomas, G. Landry, Y. Sun, R. Shubochkin, K. Balemarthy, J. Tatum, S. E. Ralph. 4
[113] S. M. R. Motaghiannezam, I. Lyubomirsky, H. Daghighian, C. Kocot, T. Gray, J. Tatum, A. Amezcua-Correa, M. Bigot-Astruc, D. Molin, F. Achten, P. Sillard. 180 Gbps PAM4 VCSEL transmission over 300 m wideband OM4 fibre. Optical Fiber Communication Conference, Th3G.2(2016).
[114] P. Kolesar. Wideband MMF standardization and S-WDM technology(2016).
[116] P.-K. Shen, C.-T. Chen, C.-H. Chang, C.-Y. Chiu, C.-C. Chang, H.-C. Lan, Y.-C. Lee, M.-L. Wu. On-chip optical interconnects integrated with laser and photodetector using three-dimensional silicon waveguides. Optical Fiber Communication Conference, M2K.6(2014).
[118] R. Santos, D. D’Agostino, F. M. Soares, H. Rabbani Haghighi, M. K. Smit, X. J. M. Leijtens. Fabrication and characterization of a wet-etched InP-based vertical coupling mirror. 18th Annual Symposium of the IEEE Photonics Benelux, 179-182(2013).
[153] J. Geng. Structured-light 3D surface imaging: a tutorial. Adv. Opt. Photon., 3, 128-160(2011).
[168] A. Liu, W. Zheng, D. Bimberg. Unidirectional transmission in finite-size high-contrast gratings. Asia Communications and Photonics Conference, AF2A.52(2016).
[193] M. Gębski, T. Czyszanowski, J. A. Lott. Electrically-injected VCSELs with a composite monolithic high contrast grating and distributed Bragg reflector coupling mirror. IEEE International Semiconductor Laser Conference (ISLC), TuP38(2018).
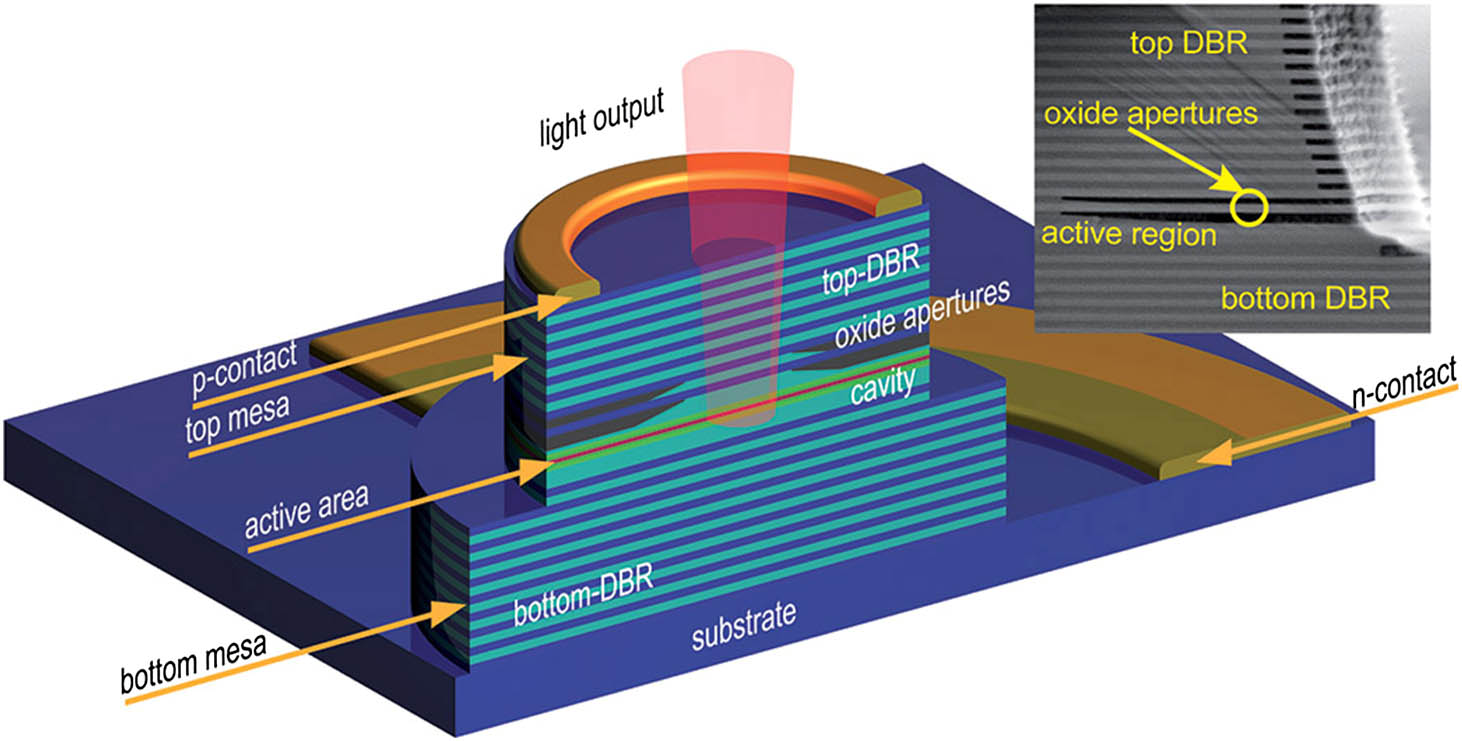
Set citation alerts for the article
Please enter your email address