Author Affiliations
1Wang Da-Heng Collaborative Innovation Center, Heilongjiang Provincial Key Laboratory of Quantum Manipulation and Control, Harbin University of Science and Technology, Harbin 150080, China2Photonics and Mathematical Optics Group, Tecnologico de Monterrey, Monterrey 64849, Mexico3School of Physics, University of the Witwatersrand, Johannesburg 2050, South Africa4Centro de Investigaciones en Óptica, A.C., Loma del Bosque 115, Colonia Lomas del campestre, 37150 León, Gto., Mexico5e-mail: b.pegar@tec.mxshow less
Fig. 1. Schematic representation of a classically entangled light mode featuring a separation of both degrees of freedom upon free space propagation. Right and left circular polarizations are represented by orange and green ellipses, while linear polarization is represented by gray lines.
Fig. 2. Phase (back panels) and polarization distribution overlapped with the intensity profiles (front panels) of the scalar modes TPG+(r;a)e^R (left) and TPG−(r;a)e^L (middle), which are combined to generate the TPGV mode (right) at the (a) near and (b) far field. In the case of the TPGV mode, we depict the phase of the complex Stokes field S=S1+iS2.
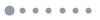
Fig. 3. (a) To experimentally generate the TPGV mode, we used a novel approach based on a digital micromirror device (DMD). A laser beam expanded and collimated (by lenses L1 and L2) is diagonally polarized with a half-wave plate (HWP1) and split by a polarizing beam splitter (PBS) according to its polarization components. The two beams are redirected to the DMD, impinging at slightly different angles but overlapped at the center of a binary multiplexed hologram where the TPG+ and TPG− scalar modes are encoded, each with a unique linear grating. After the DMD, the first diffraction order of each beam overlaps along a common propagation axis where the TPGV is generated. A spatial filter (SF) placed at the focusing plane of a telescope formed by lenses L3 and L4 removes all higher diffraction orders. A quarter-wave plate (QWP1) transforms the mode from the linear to the circular polarization basis. The nonseparability dynamics are quantified through Stokes polarimetry, for which a set of four intensities are recorded with a charge-coupled device (CCD) camera. (b) Experimental Stokes parameters S1, S2, and S3 of the TPGV mode TPGV(r,3). (c) Intensity profile overlapped with the reconstructed polarization distribution.
Fig. 4. (a) Experimental and (b) simulated evolution of intensity and polarization distribution of TPGV beams as a function of propagation distance. (c) Experimental and (d) simulated representation of the transverse polarization distribution on a Poincaré sphere, each in correspondence with the planes shown in (a) and (b).
Fig. 5. (a) Concurrence as a function of propagation and transverse coordinates. The solid and dashed lines show two examples of
C as a function of
z for the specific sections of the beam, shown in (b) and (c), which correspond to
z=0 and
z=4zmax, respectively. The surface corresponds to numerical simulations and the data points to experimental measurements at the propagation planes
z/zmax=0, 0.2, 0.7, 1.3, 2.2, and 3.6. An analogous behavior has been reported in the context of temporal coherence [
58], where the generation of beams whose local degree of temporal coherence varies as a function of the time difference is discussed.