Peihang Li, Peng Yu, Jiachen Sun, Zhimin Jing, Jiang Wu, Lucas V. Besteiro, Roberto Caputo, Arup Neogi, Hongxing Xu, Zhiming Wang, "Directional radiation enhancement of nanowire quantum dots based on line-array plasmonic antenna coupling," Photonics Res. 10, 2178 (2022)

Search by keywords or author
- Photonics Research
- Vol. 10, Issue 9, 2178 (2022)
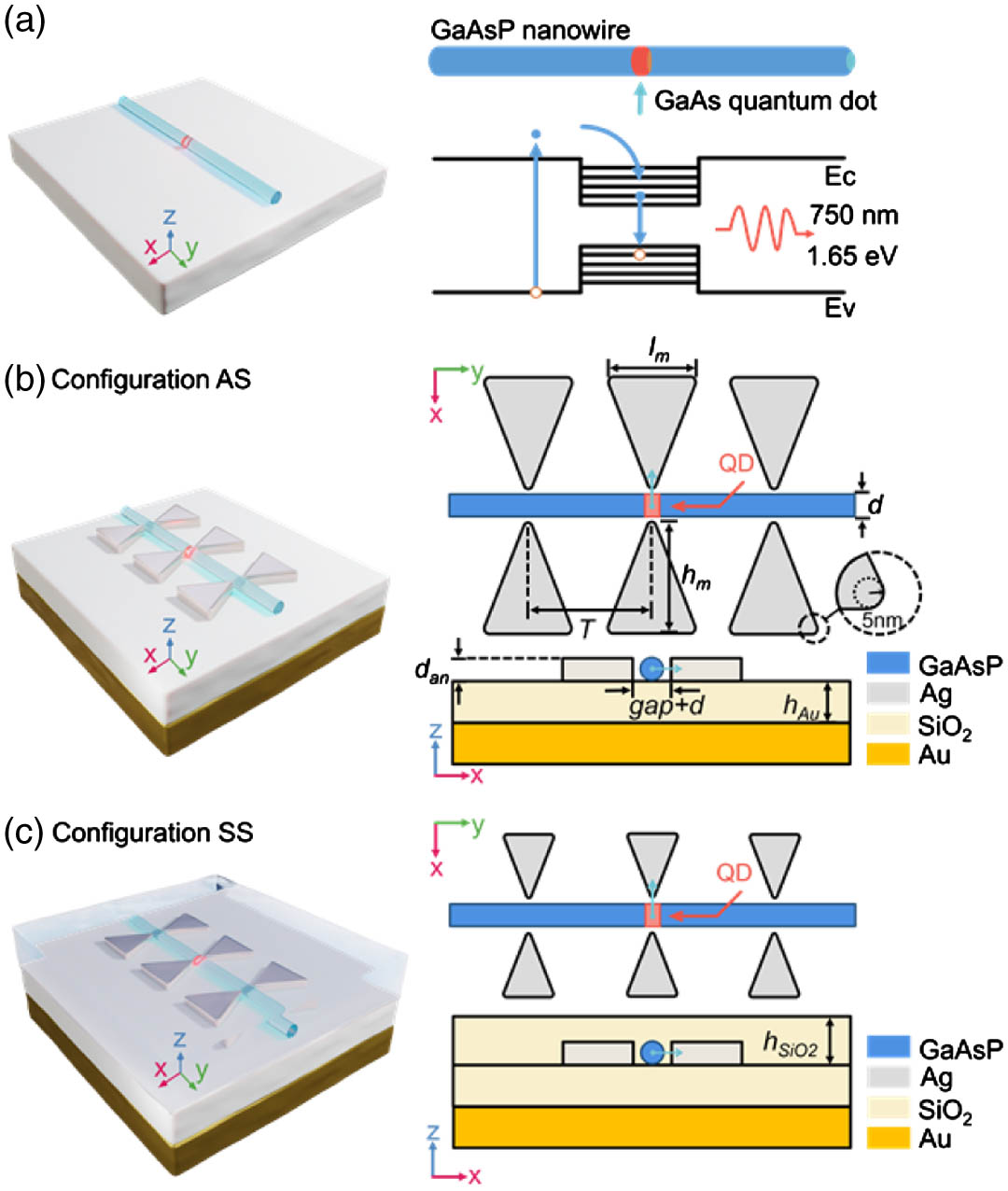
Fig. 1. (a) Schematic of the NWQD (left); schematic energy band diagram for the QD embedded in a nanowire (right). (b) Configuration AS, NWQD coupled to a line-array plasmonic bowtie nanoantenna in an air superstrate with a gold mirror. (c) Configuration SS, NWQD coupled to a line-array plasmonic bowtie nanoantenna in a SiO 2 superstrate with a gold mirror. The dipole moment of the QD points along the bowtie antenna axis; the chamfer of the bowtie is set to 5 nm. The d is consistent with the nanowire diameter and gap = 15 nm , d an = 20 nm . The origin of the coordinate system locates at the center of the QD.
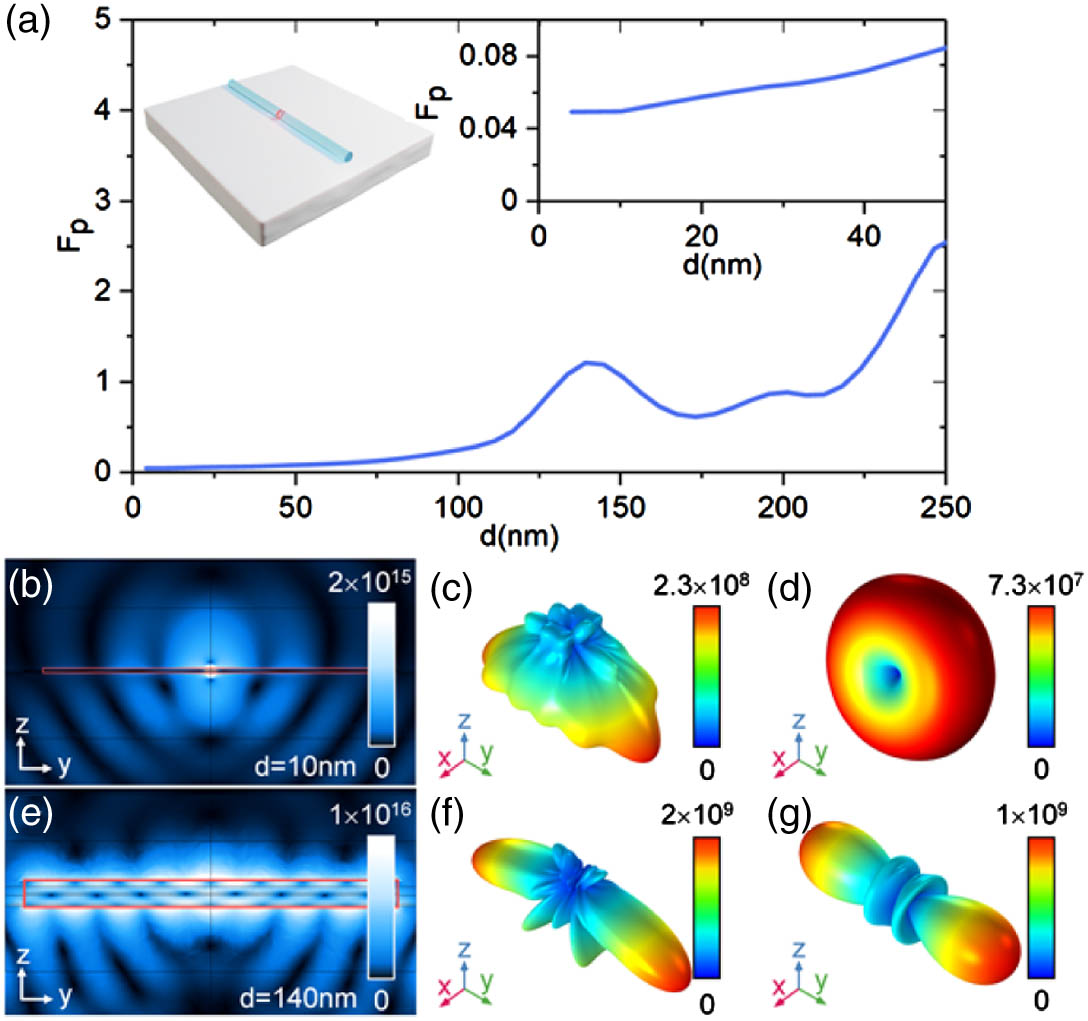
Fig. 2. (a) Trend of the variation of F p with a variation of the GaAsP/GaAs NWQD’s diameter d . (b), (c) Electric field modulus distributions (V/m) and far-field radiation pattern (V/m) of the NWQD with d = 10 nm at the substrate, respectively; the red outline is the NWQD. (d) Far-field radiation pattern (V/m) of the NWQD with d = 10 nm in free space. (e), (f) Electric field modulus distributions (V/m) and far-field radiation pattern (V/m) of the NWQD with d = 140 nm at the substrate, respectively; the red outline is the NWQD. (g) Far-field radiation pattern (V/m) of the NWQD with d = 140 nm in free space.
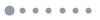
Fig. 3. (a) The NWQD coupled to a single plasmonic bowtie nanoantenna. (b) F p , P L of the NWQD coupled to a single plasmonic bowtie nanoantenna change with l m and h m at d = 10 nm , d an = 20 nm . (c) The far-field radiation pattern of the NWQD is coupled to a single plasmonic bowtie nanoantenna at the substrate. (d) The far-field radiation pattern of the NWQD is coupled to a single plasmonic bowtie nanoantenna in free space.
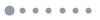
Fig. 4. (a) The NWQD coupled to a line-array plasmonic bowtie nanoantenna. (b) F p , P L , and the P L ratio of the NWQD coupled to a line-array plasmonic bowtie nanoantenna change with T . The inset is far-field patterns for different T . (c), (d) Electric field modulus distributions (V/m) and phase distribution of the NWQD coupled to a line-array plasmonic bowtie nanoantenna. The red outline is the NWQD, and the three red dots represent the tips of the three bowties. (e) Far-field radiation pattern (V/m) of the NWQD coupled to a line-array plasmonic bowtie nanoantenna at T = 420 nm .
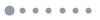
Fig. 5. (a) Electric field mode distributions of three pairs and a single plasmonic antenna under plane wave excitation. (b) Schematic diagram of single QD–line-array plasmonic antennas-coupled device. (c) Electric field mode distribution (V/m) and far-field radiation pattern (V/m) of single QD–line-array plasmonic antennas-coupled device, and the three red dots represent the tips of the three bowties.
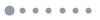
Fig. 6. (a) Schematic diagram of a simplified mathematical model of the line-array antenna. (b) Main lobe directivity of the far-field radiation pattern of the simplified mathematical model of the line-array antenna. The inset is far-field patterns for different T .
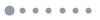
Fig. 7. (a) F p , P L , and the P L ratio of the AS with different h Au . (b), (c) The electric field modulus distribution (V/m) and far-field radiation pattern (V/m) of the AS when h Au = 560 nm . The red outline is the NWQD, and the three red dots represent the tips of the three bowties.
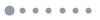
Fig. 8. (a) F p , P L , and the P L ratio of the SS with different h Au . (b), (c) The electric field modulus distribution (V/m) and far-field radiation pattern (V/m) of the SS when h Au = 560 nm . The red outline is the NWQD, and the three red dots represent the tips of the three bowties.
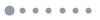
Fig. 9. Schematic diagram of the structure used in the physics simulation. Perfectly matched layers are placed outside the physical domain to simulate the propagation of electromagnetic waves in free space (absorbing electromagnetic waves to prevent reflected waves from affecting the structure itself).
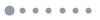
Fig. 10. Influence of relative distance between the plasmonic antenna and the QD on enhancement effect.
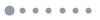
Fig. 11. Distribution of electric field film (V/m) of five-pair plasmon antenna–NWQD coupling structure. Five-pair plasmon antenna–NWQD coupling structure electric field film distribution. The red outline is the NWQD, and the five red dots represent the tips of the three bowties.
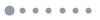
Fig. 12. Effect of the relative errors of the NWQD and plasmonic antenna positions in the AS.
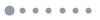
Fig. 13. Effect of the relative errors of the NWQD and plasmonic antenna positions in the SS.
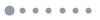
Fig. 14. Collection efficiency of the SS with a different numerical aperture (NA).
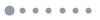
Fig. 15. Collection efficiency of the AS with a different numerical aperture (NA).
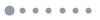
Fig. 16. Performance effect of h SiO 2 on the SS without a gold mirror. The inset is a schematic cross section of the device.
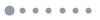
Fig. 17. Far-field radiation pattern of the SS without gold mirrors with different h SiO 2 . (a) Far-field radiation pattern at h SiO 2 = 40 nm . (b) Far-field radiation pattern at h SiO 2 = 80 nm . (c) Far-field radiation pattern at h SiO 2 = 160 nm . (d) Far-field radiation pattern at h SiO 2 = 240 nm .
|
Table 1. Comparison of SPE
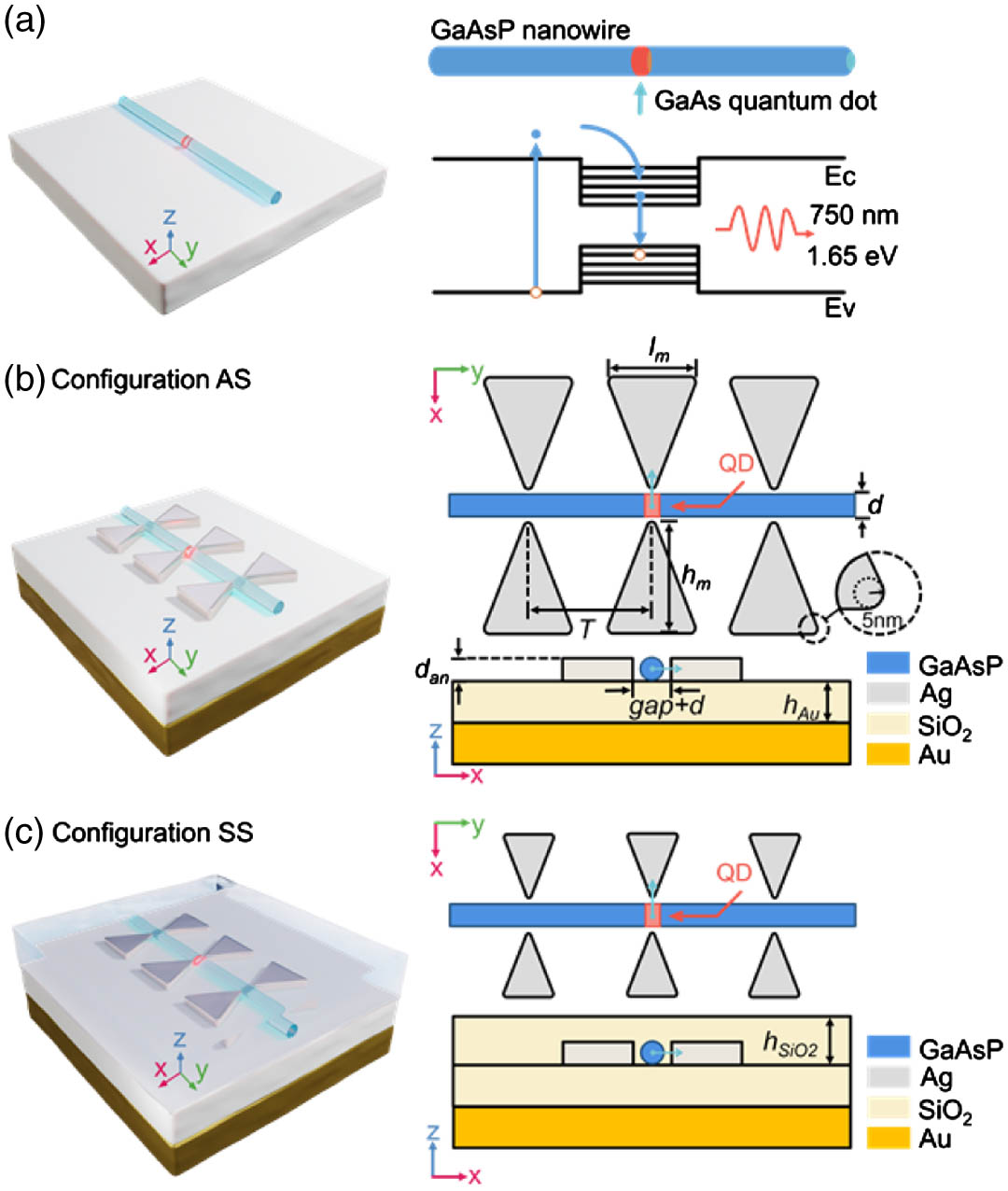
Set citation alerts for the article
Please enter your email address