Bin Dong, Juan Zhang, Dawei Wang, Yiyuan Zhang, Leran Zhang, Rui Li, Chen Xin, Shunli Liu, Zihang Zhang, Hao Wu, Shaojun Jiang, Suwan Zhu, Bingrui Liu, Dong Wu. Femtosecond laser micromachining optical devices[J]. Opto-Electronic Engineering, 2023, 50(3): 220073

Search by keywords or author
- Opto-Electronic Engineering
- Vol. 50, Issue 3, 220073 (2023)
![Schematic diagram of the super-resolution processing principle and characteristics after processing[4]. Figure reproduced with permission from ref. [4] © Springer Nature](/richHtml/gdgc/2023/50/3/220073/4_220073-1.jpg)
Fig. 1. Schematic diagram of the super-resolution processing principle and characteristics after processing[4]. Figure reproduced with permission from ref. [4] © Springer Nature
![Femtosecond laser direct writing[45]. Figure reproduced with permission from ref. [45] © De Gruyter](/richHtml/gdgc/2023/50/3/220073/4_220073-2.jpg)
Fig. 2. Femtosecond laser direct writing[45]. Figure reproduced with permission from ref. [45] © De Gruyter
![Schematic diagram of the femtosecond laser fabrication based on diffractive optical elements and characteristics after processing[49]. Figure reproduced with permission from ref. [49] © Springer Nature](/Images/icon/loading.gif)
Fig. 3. Schematic diagram of the femtosecond laser fabrication based on diffractive optical elements and characteristics after processing[49]. Figure reproduced with permission from ref. [49] © Springer Nature
![The schematic diagram of the digital micromirror projection system based on femtosecond laser two-photon polymerization and spatio-temporal focusing and characteristics after processing[57]. Figure reproduced with permission from ref. [57] © The American Association for the Advancement of Science(AAAS)](/Images/icon/loading.gif)
Fig. 4. The schematic diagram of the digital micromirror projection system based on femtosecond laser two-photon polymerization and spatio-temporal focusing and characteristics after processing[57]. Figure reproduced with permission from ref. [57] © The American Association for the Advancement of Science(AAAS)
![Parallel processing based on spatial light modulator. (a) Helical scanning of femtosecond ring-shaped vortex beam based on spatial light modulator[65]; (b) Generation of Mathieu beam based on spatial light modulator[66]; (c) Fabrication of microtubes using C-shaped Bessel beam generated by spatial light modulator[68]. Figure reproduced with permission from: (a) ref. [65] © Wiley; (b) ref. [66] and (c) ref. [68] © American Chemical Society.](/Images/icon/loading.gif)
Fig. 5. Parallel processing based on spatial light modulator. (a) Helical scanning of femtosecond ring-shaped vortex beam based on spatial light modulator[65]; (b) Generation of Mathieu beam based on spatial light modulator[66]; (c) Fabrication of microtubes using C-shaped Bessel beam generated by spatial light modulator[68]. Figure reproduced with permission from: (a) ref. [65] © Wiley; (b) ref. [66] and (c) ref. [68] © American Chemical Society.
![Focal field engineering based on spatial light modulator[72]. Figure reproduced with permission from ref. [72] © John Wiley and Sons](/Images/icon/loading.gif)
Fig. 6. Focal field engineering based on spatial light modulator[72]. Figure reproduced with permission from ref. [72] © John Wiley and Sons
![Schematic diagram of the femtosecond (fs) laser interference processing and characteristics after processing[79]. Figure reproduced with permission from ref. [79] © MDPI](/Images/icon/loading.gif)
Fig. 7. Schematic diagram of the femtosecond (fs) laser interference processing and characteristics after processing[79]. Figure reproduced with permission from ref. [79] © MDPI
![Processing of microlenses. (a) Transferring[83]; (b) Wet etching[84]; (c) Dry etching[86]. Figure reproduced with permission from: (a) ref. [83], (b) ref. [84] and (c) ref. [86] © Wiley](/Images/icon/loading.gif)
Fig. 8. Processing of microlenses. (a) Transferring[83]; (b) Wet etching[84]; (c) Dry etching[86]. Figure reproduced with permission from: (a) ref. [83], (b) ref. [84] and (c) ref. [86] © Wiley
![Micromachining of microlenses based on two-photon polymerization of femtosecond laser. (a) Protein-based microlens in response to the change of pH[93]; (b) Double-material compound eye in response to the change of pH[25]. Figure reproduced with permission from: (a) ref. [93] and (b) ref. [25] © Wiley](/Images/icon/loading.gif)
Fig. 9. Micromachining of microlenses based on two-photon polymerization of femtosecond laser. (a) Protein-based microlens in response to the change of pH[93]; (b) Double-material compound eye in response to the change of pH[25]. Figure reproduced with permission from: (a) ref. [93] and (b) ref. [25] © Wiley
![Innovative microlenses. (a) Complex microlens arrays based on laser-induced thermal deformation[95]; (b) Direct patterning of polymeric microlenses based on the combination of femtosecond laser and surface tension of droplet[96]; (c) Integration of compound lens on the surface of CMOS image sensor based on femtosecond laser[82]. Figure reproduced with permission from: (a) ref.[95] © Springer Nature; (b) ref. [96] © American Chemical Society; (c) ref. [82] © The American Association for the Advancement of Science (AAAS)](/Images/icon/loading.gif)
Fig. 10. Innovative microlenses. (a) Complex microlens arrays based on laser-induced thermal deformation[95]; (b) Direct patterning of polymeric microlenses based on the combination of femtosecond laser and surface tension of droplet[96]; (c) Integration of compound lens on the surface of CMOS image sensor based on femtosecond laser[82]. Figure reproduced with permission from: (a) ref.[95] © Springer Nature; (b) ref. [96] © American Chemical Society; (c) ref. [82] © The American Association for the Advancement of Science (AAAS)
![Femtosecond laser direct writing optical waveguide. (a) Single-line waveguide[100]; (b) Double-line waveguide[106]; (c) Depressed cladding waveguide[111]. Figure reproduced with permission from: (a) ref. [100] and (c) ref. [111] © Wiley; (b) ref. [106] © MDPI.](/Images/icon/loading.gif)
Fig. 11. Femtosecond laser direct writing optical waveguide. (a) Single-line waveguide[100]; (b) Double-line waveguide[106]; (c) Depressed cladding waveguide[111]. Figure reproduced with permission from: (a) ref. [100] and (c) ref. [111] © Wiley; (b) ref. [106] © MDPI.
![Fabrication of micro-gratings based on femtosecond laser. (a) Procedures of femtosecond laser fabricating FBG[117]; (b) Fabrication of LIPSS on Si substrate utilizing cylindrical lens based on femt osecond laser[129]; (c) Fabrication of continuous phase vortex grating based on two-photon polymerization of femtosecond laser[22]. Figure reproduced with permission from: (a) ref. [117] © American Chemical Society; (b) ref. [129] © Wiley; (c) ref. [22] © AIP Publishing](/Images/icon/loading.gif)
Fig. 12. Fabrication of micro-gratings based on femtosecond laser. (a) Procedures of femtosecond laser fabricating FBG[117]; (b) Fabrication of LIPSS on Si substrate utilizing cylindrical lens based on femt osecond laser[129]; (c) Fabrication of continuous phase vortex grating based on two-photon polymerization of femtosecond laser[22]. Figure reproduced with permission from: (a) ref. [117] © American Chemical Society; (b) ref. [129] © Wiley; (c) ref. [22] © AIP Publishing
![Fabrication of woodpile photonic crystal whose stop band lies in the visible spectrum based on femtosecond laser[146]. Figure reproduced with permission from ref. [146] © Springer Nature](/Images/icon/loading.gif)
Fig. 13. Fabrication of woodpile photonic crystal whose stop band lies in the visible spectrum based on femtosecond laser[146]. Figure reproduced with permission from ref. [146] © Springer Nature
![Fabrication of nonlinear photonic crystal based on femtosecond laser. (a) Fabrication of nonlinear photonic crystal in LiNbO3[150]; (b) Fabrication of nonlinear photonic crystal in BCT[151]. Figure reproduced with permission from: (a) ref. [150] and (b) ref. [151] © Springer Nature](/Images/icon/loading.gif)
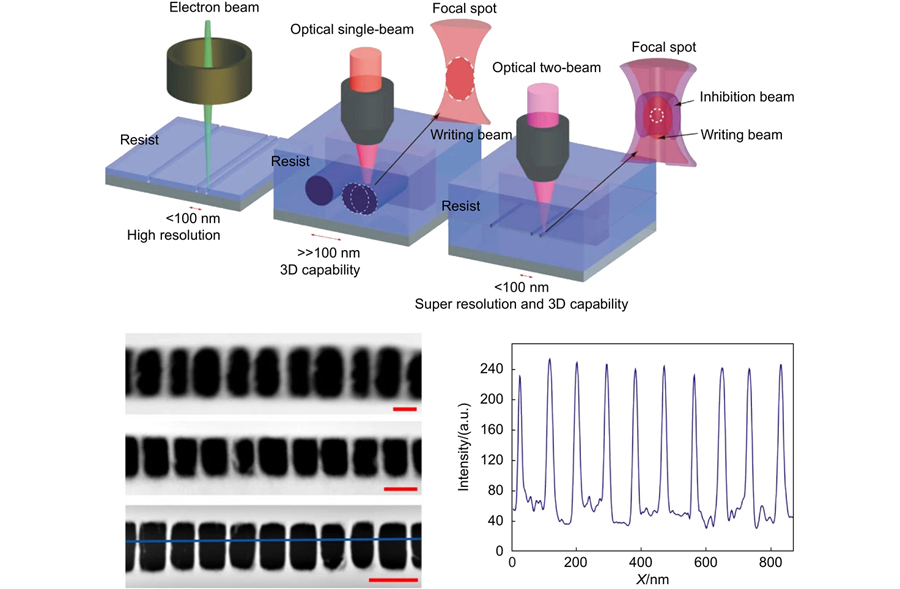
Set citation alerts for the article
Please enter your email address