Author Affiliations
1State Key Laboratory of Applied Optics, Changchun Institute of Optics, Fine Mechanics and Physics, Chinese Academy of Sciences, Changchun 130033, China2University of Chinese Academy of Sciences, Beijing 100049, Chinashow less
Fig. 1. (a) Lineshape comparison between Lorentz (red line) and Fano (blue dotted line) with the same Q-factor. (ω0,T0) is the extremum of the Lorentzian dip, and (ω1,T1), (ω2,T2) are the two extrema of Fano. (b) Schematic diagram of the add-drop coupling configuration used here and its optical spectral responses. κext and κexd denote the coupling rates of the in-through and add-drop fibers with the resonator, respectively. If there is Rayleigh-scattering-induced unresolvable mode splitting, the add port shows an upward Lorentzian peak.
Fig. 2. (a) Dependence of the Fano profile on phase difference φ0. The black dot denotes the maximum and the gray dot denotes the minimum. The extrema far away from the zero-detuning point are lost due to the limited plot range. (b) Fitted experimental results of a Fano periodical evolution. The fitting parameters are κ0=0.14 MHz and Ec0:αin=1.6. κext=1.92 MHz and κexd=2.4 MHz are uniquely determined by fitting the transmission spectrum and the Fano profile simultaneously. The Q-factor of this resonance is approximately 4.34×107. (c), (d) Dependence of the frequency shift and intensity magnitude of the maximum, the minimum, and their difference on the amplitude ratio when φ0=8π/5. This phase is chosen as an example to illustrate the dependence, and for other phases except the integral multiple of π, the key points show similar dependence.
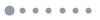
Fig. 3. (a)–(d) Transmitting (yellow) and back-scattering (green) spectral responses for different relative phases φ0. Solid lines are experimental results, and dotted lines are theoretical fittings. The mismatch is attributed to the laser power fluctuation and background modification induced by the fiber connectors. The parameters used in fitting are κ0=10.8 MHz, g=0.52 MHz, Γ=0.18 MHz, Ec0=0.985 normalized to the input field intensity, and κexd=3.7 MHz and κext=15.2 MHz are slightly changed due to vibration-induced coupling condition changes. The Q-factors are approximately 6.43×106 for the symmetrical mode and 6.51×106 for the asymmetrical mode, respectively. Note that to achieve an unresolved doublet caused by a machining defect, a new microsphere resonator with a similar diameter was utilized. (e) Back-scattering spectral responses for different coherent field intensities (0.138, 0.196, and 0.285 normalized to the input field intensity) with a relative phase φ0 around 0.5π. The profile becomes easily identifiable and immune against noise as the intensity increases. (f) Dependence of the intensity difference |Tmax−Tmin| on the amplitude ratio. The red dotted line is the theoretical result using the experimental parameters.
Fig. 4. Frequency difference between the two extrema of the Fano-based doublet for φ0=0. Here 2g starts from 0.2 MHz, Γ=0.05g, and κext equals 0.4, 14.5, and 20 for under-, critical-, and over-coupling conditions, respectively, and other parameters are the same as in the experiment. The fitting curves are 8.56+0.061×(2g)1.787, 16.66+0.037×(2g)1.781, and 19.82+0.032×(2g)1.781, respectively. The length of the fitting curve denotes the range 2g≤Γ+κext+κext+κ0 for each condition.