Weiguang Liu, Bin Hu, Zongduo Huang, Hongyu Guan, Heting Li, Xinke Wang, Yan Zhang, Hongxing Yin, Xiaolu Xiong, Juan Liu, Yongtian Wang, "Graphene-enabled electrically controlled terahertz meta-lens," Photonics Res. 6, 703 (2018)

Search by keywords or author
- Photonics Research
- Vol. 6, Issue 7, 703 (2018)
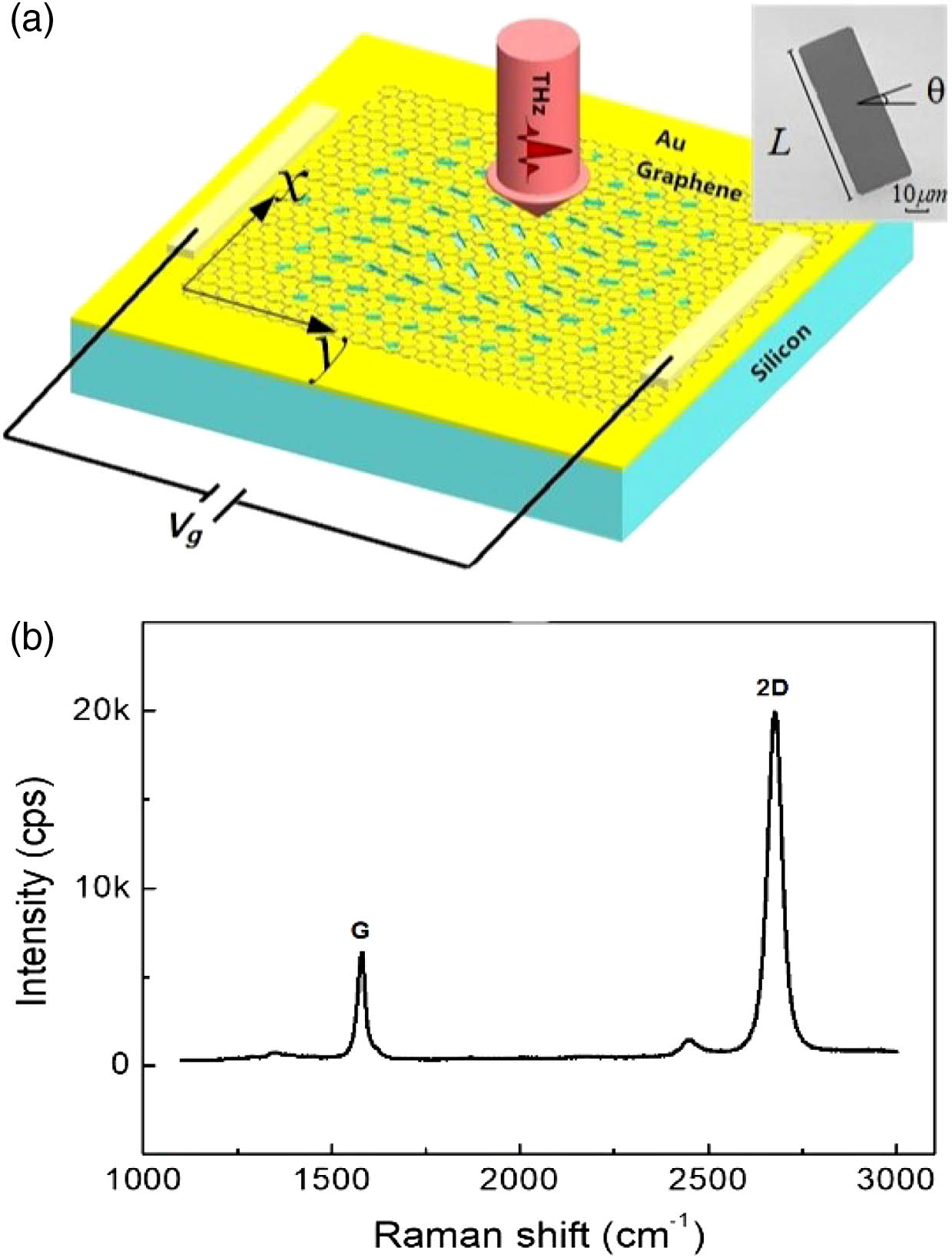
Fig. 1. (a) Schematic of the active THz meta-lens. The lens consists of a high-resistance silicon substrate, an Au metasurface of rectangular aperture antennas with different lengths and rotations, and a monolayer graphene. The incident THz wave is left-handed circularly polarized. The inset shows an SEM photograph of a unit cell of the apertures. (b) Raman spectrum of the monolayer graphene on the top of the Au metasurface.
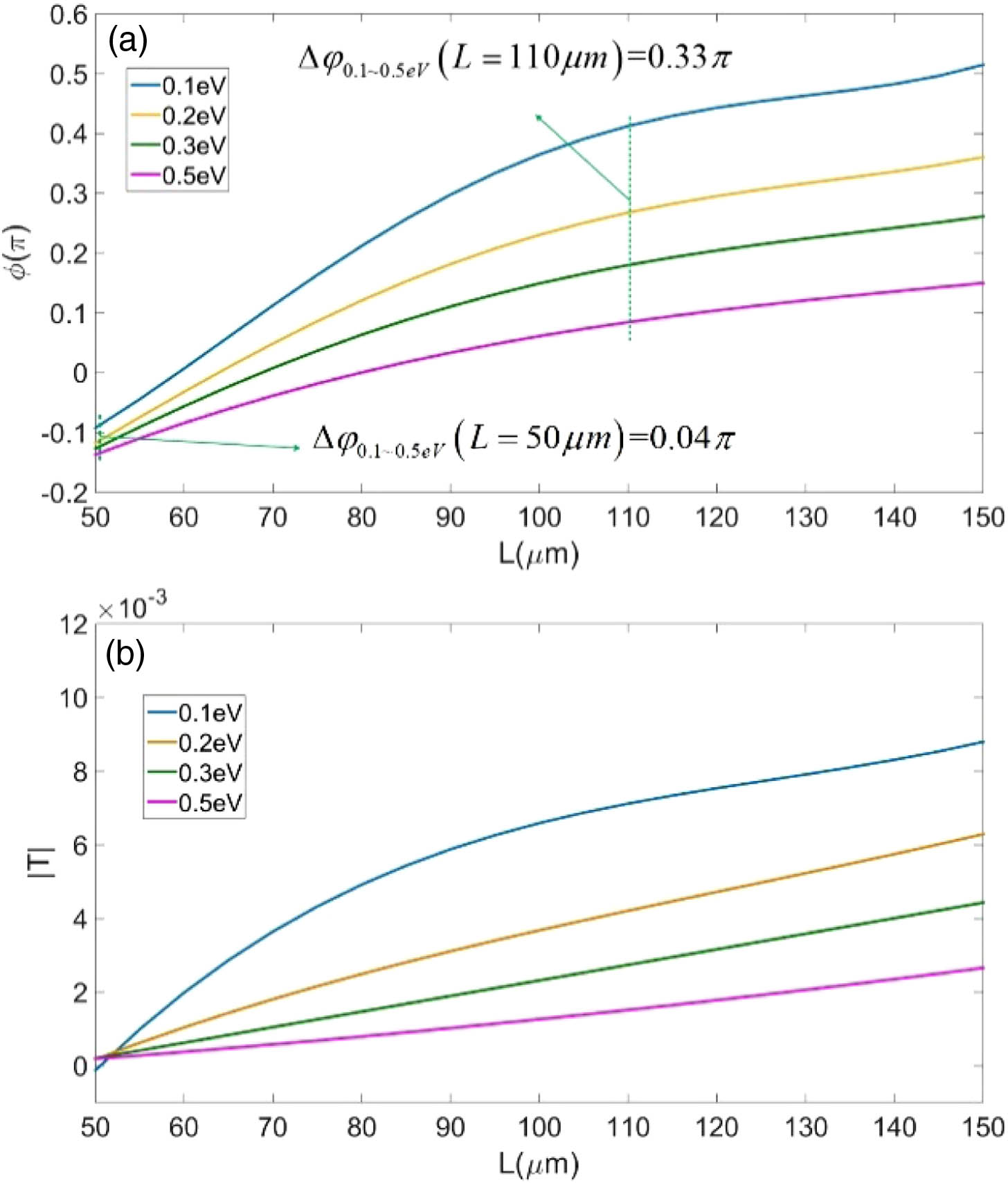
Fig. 2. Dependence of transmitted RCP THz wave on the aperture length and graphene chemical potential. The aperture width is fixed as 20 μm. (a) Phase change of RCP THz wave as a function of L , when E F changes from 0.1 to 0.5 eV. (b) Transmission of RCP THz wave as a function of L , when E F changes from 0.1 to 0.5 eV.
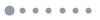
Fig. 3. Design of the meta-lens. The focal length is designed to increase from 7.0 to 9.0 mm, when E F changes from 0.1 to 0.5 eV. (a) The corresponding phase distributions (blue lines) of focal lengths are 7.0 and 9.0 mm, respectively, and the phase difference (red line) between them is shown. Phase distribution as a function of the aperture length dependent on the graphene chemical potential. (b) Length and (c) rotation angle distributions of the apertures of the proposed tunable lens.
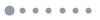
Fig. 4. (a) Fabrication process of the electrically tunable THz meta-lens. (b) SEM micrograph of the fabricated device.
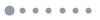
Fig. 5. Experimental results of the tunable meta-lens. (a) and (b) Measured transmitted RCP THz wave intensity (normalized) and phase (rad) distributions of the imaging plane with a distance of 2.0 mm behind the meta-lens when V g = 0.0 V , respectively. (c) and (d) Measured intensity (normalized) and phase (rad) distributions of the imaging plane when V g = 2.0 V , respectively. (e) and (f) Calculated far-field intensity distribution of RCP THz wave based on the measured intensity and phase of the imaging plane when V g = 0.0 V and V g = 2.0 V , respectively. The frequency of the incident THz wave is 0.75 THz.
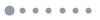
Fig. 6. Simulation results of the active lens. (a) Gate-dependent electrical resistance of the graphene on the metasurface. (b) and (c) FDTD simulations of far-field intensity distribution of RCP THz wave when E F 1 = 0.15 eV and E F 2 = 0.42 eV , respectively.
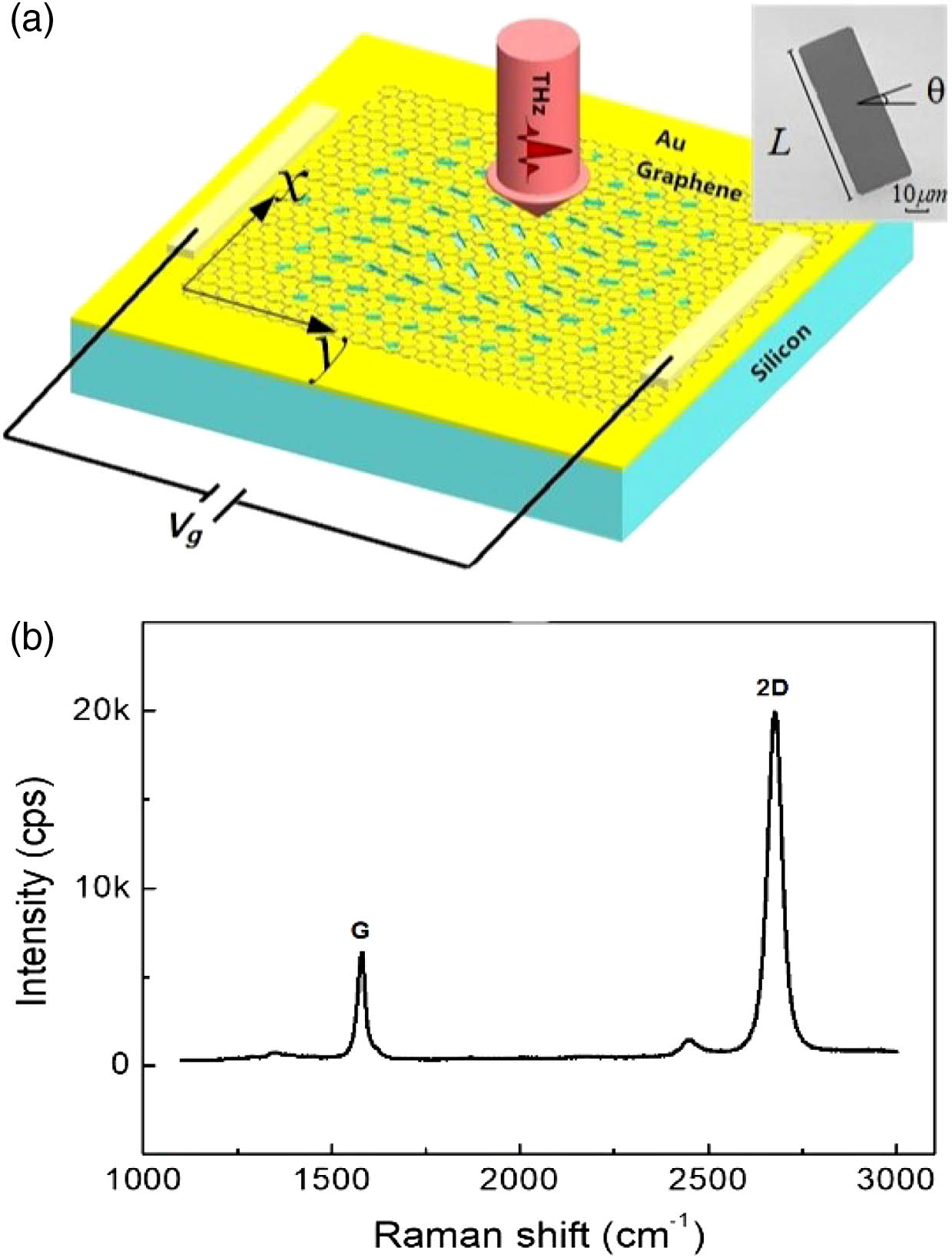
Set citation alerts for the article
Please enter your email address