Yingying Zhang, Shiqiang Xia, Xingdong Zhao, Lu Qin, Xuejing Feng, Wenrong Qi, Yajing Jiang, Hai Lu, Daohong Song, Liqin Tang, Zunlue Zhu, Wuming Liu, Yufang Liu, "Symmetry-protected third-order exceptional points in staggered flatband rhombic lattices," Photonics Res. 11, 225 (2023)

Search by keywords or author
- Photonics Research
- Vol. 11, Issue 2, 225 (2023)
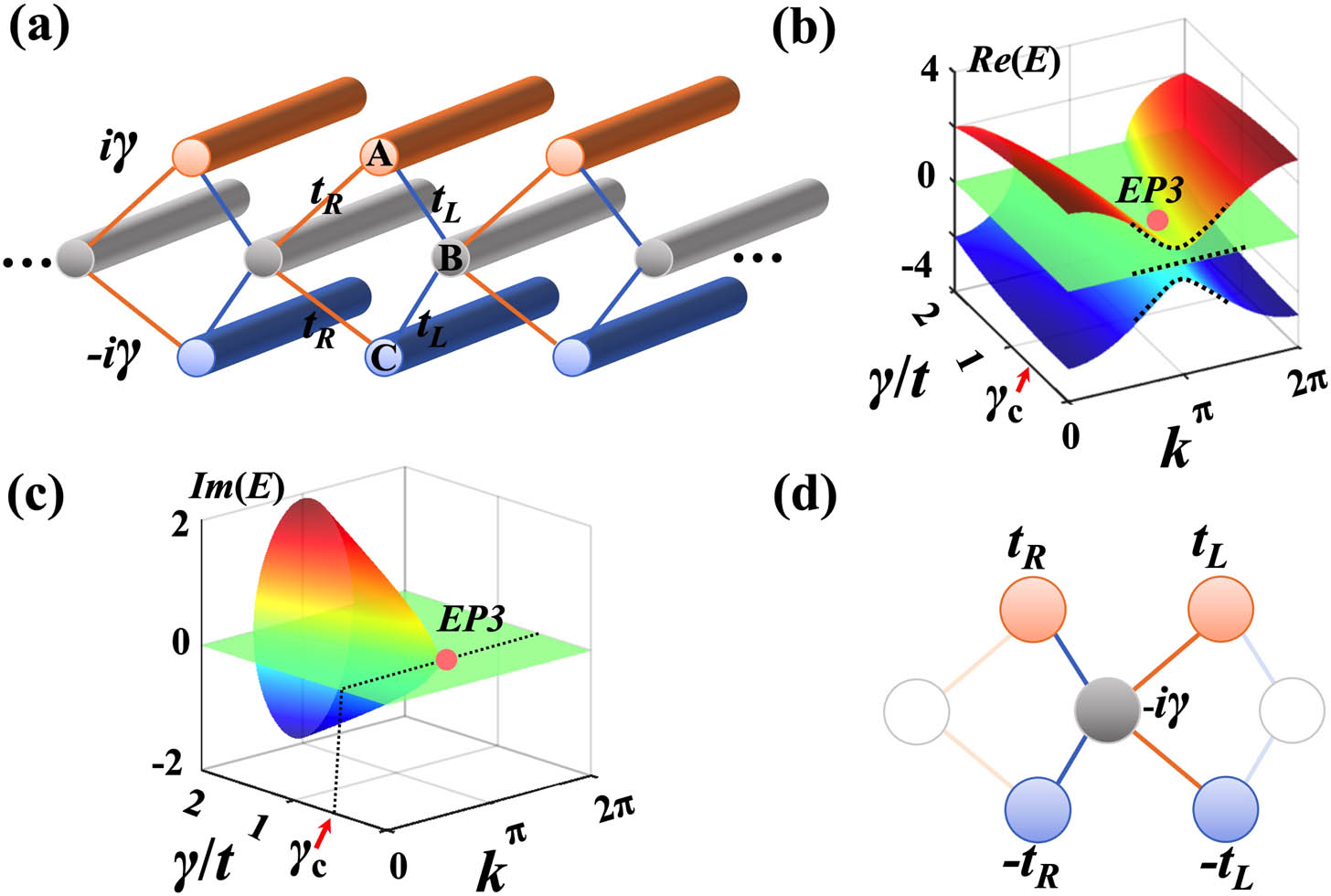
Fig. 1. (a) Schematic of the diagonal PT -symmetric photonic rhombic lattices formed by waveguide arrays without non-Hermitian coupling. The unit cell consists of three sites: two edge sites with gain γ (A , red) or loss − γ (C , blue), and one central site with a neutral imaginary part (B , gray). The staggered coupling coefficients are t L = t ( 1 + g ) and t R = t ( 1 − g ) , where 0 ≤ g < 1 and t is the coupling coefficient of uniform lattices (g = 0 ). For simplicity, we set the lattice period l = 1 . (b), (c) Calculated real (b) and imaginary (c) parts of the spectrum as a function of γ / t for g = 0.2 . The red circles represent the position of the EP3 and the corresponding critical value of phase transition is γ c / t = 0.56 . (d) Field distribution of a flatband CLS, which occupies two unit cells. The characters show the corresponding amplitudes.
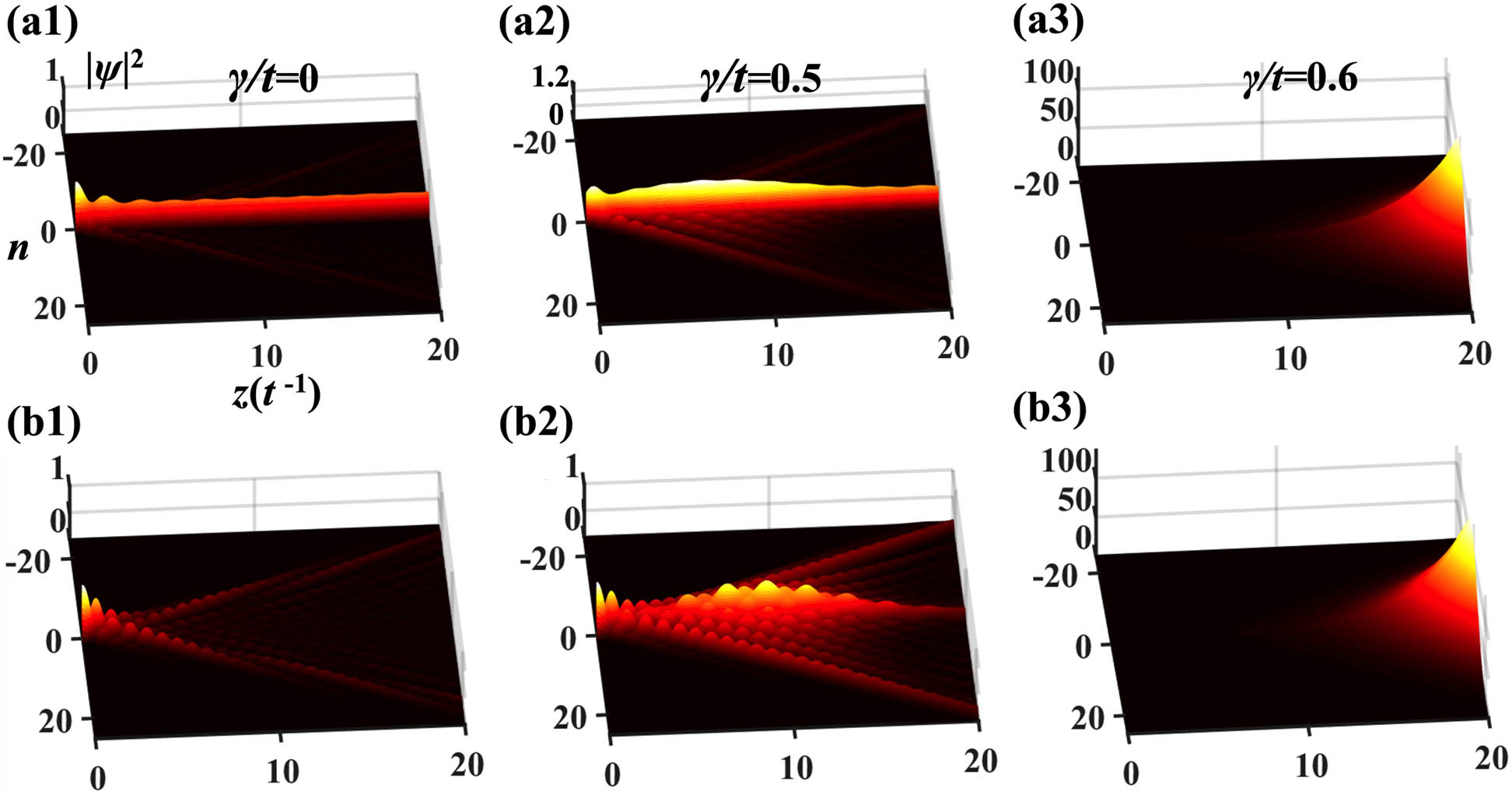
Fig. 2. Intensity profile | ψ | 2 of a single-site A (a1)–(a3) or B (b1)–(b3) excitation. (a1) The A sublattice excitation for Hermitian case (γ / t = 0 ) exhibits a suppression of diffraction due to the excitation of flatband CLSs. (a2) The input remains conserved below the EP3 threshold (γ / t = 0.5 ). (a3) The input leads to an exponentially growing total intensity in the PT -broken phase (γ / t = 0.6 ). (b1) The B site excitation for Hermitian case (γ / t = 0 ), showing a typically discrete diffraction pattern. (b2) The input excites both the flatband and dispersive bands, producing discrete diffraction as well as a residual localized component (γ / t = 0.5 ). (b3) The input shows same exponential growth of total intensity as in (a3) (γ / t = 0.6 ). The propagation length is z = 20 t − 1 . Note the different scales of the vertical axis in these panels, though the initial amplitudes of the excitation all equal 1.
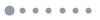
Fig. 3. (a), (b) Analytical (dashed lines) and numerical (solid lines) results for the (a) real and (b) imaginary parts of the spectrum as a function of the detuning ϵ / t in the vicinity of EP3. Red lines, E − 1 ; green lines, E 0 ; blue lines, E 1 . (c) Corresponding results for E 0 − E 1 = Δ E , exhibiting a cube-root behavior; (d) results from (c) on a logarithmic scale. The slope of about 1/3 confirms the cube-root response.
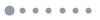
Fig. 4. (a), (b) Intensity profile for the B site excitation in the presence of small perturbations ϵ / t = 0.03 and ϵ / t = 0.06 , respectively. For ϵ / t = 0.03 , a similar pattern compared with Fig. 2 (b2) is obtained. (c), (d) Same as (a), (b) but for the excitation of a flatband CLS. The CLS can stay well localized despite the presence of the lattice perturbations.
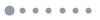
Fig. 5. (a) Schematic of the off-diagonal PT -symmetric photonic rhombic lattices formed by waveguide arrays with non-Hermitian coupling. The complex-coupling coefficients are T L = t L + i γ , T L * = t L − i γ , T R = t R + i γ , and T R * = t R − i γ , respectively. Note that the real parts (t L and t R ) are the same as in Fig. 1 (a), which represent conserved staggered couplings. (b), (c) Calculated (b) real and (c) imaginary parts of the spectrum as a function of γ / t for g = 0.2 ; the EP3 forms at k = 0 and the critical value of the phase transition is γ c = t . (d) Field distribution of the flatband CLS, which also occupies two unit cells but only distributes in A and C sites.
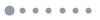
Fig. 6. Same as Fig. 2 , but for the off-diagonal PT -symmetric lattices. (a1) The A sublattice excitation oscillates periodically below the EP3 threshold (γ / t = 0.45 ). Most of the energy stays localized due to the excitation of the flatband. (a2) The quasi-energy is conserved, and the oscillation period is doubled for γ / t = 0.9 . (a3) The input leads to quartic total intensity growth once the system is operated in the PT symmetry-broken phase (γ / t = 1.001 ). (b1) The B input only excites eigenstates of the dispersive bands and therefore experiences conventional diffraction. (b2) The spreading velocity diminishes with the increase of γ / t . (b3) The input becomes localized, and the total intensity also show a quartic increase in the PT symmetry-broken phase.
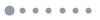
Fig. 7. Same as Fig. 3 , but for the off-diagonal PT -symmetric lattices.
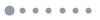
Fig. 8. Same as Fig. 4 , but for the off-diagonal PT -symmetric lattices.
![Energy evolution in the symmetry-broken phase of the (a) diagonal and (b)–(d) off-diagonal PT-symmetric lattices. (a) The power increase for the excitation of A and B sublattices is identical and follows an exponential amplification [P(z)=e0.39z]. (b)–(d) The power increases for the excitation of (b) A and (c) B sublattices follow quartic amplifications [P(z)∼z4], and the corresponding intensities P1/4 increase linearly. Note that the two lines in (d) have different slopes.](/Images/icon/loading.gif)
Fig. 9. Energy evolution in the symmetry-broken phase of the (a) diagonal and (b)–(d) off-diagonal PT -symmetric lattices. (a) The power increase for the excitation of A and B sublattices is identical and follows an exponential amplification [ P ( z ) = e 0.39 z ] . (b)–(d) The power increases for the excitation of (b) A and (c) B sublattices follow quartic amplifications [ P ( z ) ∼ z 4 ] , and the corresponding intensities P 1 / 4 increase linearly. Note that the two lines in (d) have different slopes.
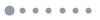
Fig. 10. (a) Intensity profile | ψ | 2 of a localized B sublattice excitation for γ / t = 0.98 . When γ / t approaches the EP3, the diffraction is prevented. (b) Behavior of the spreading velocity ν as a function of γ / t , computed for the largest propagation length z = 20 t − 1 ; ν is expressed in units of t .
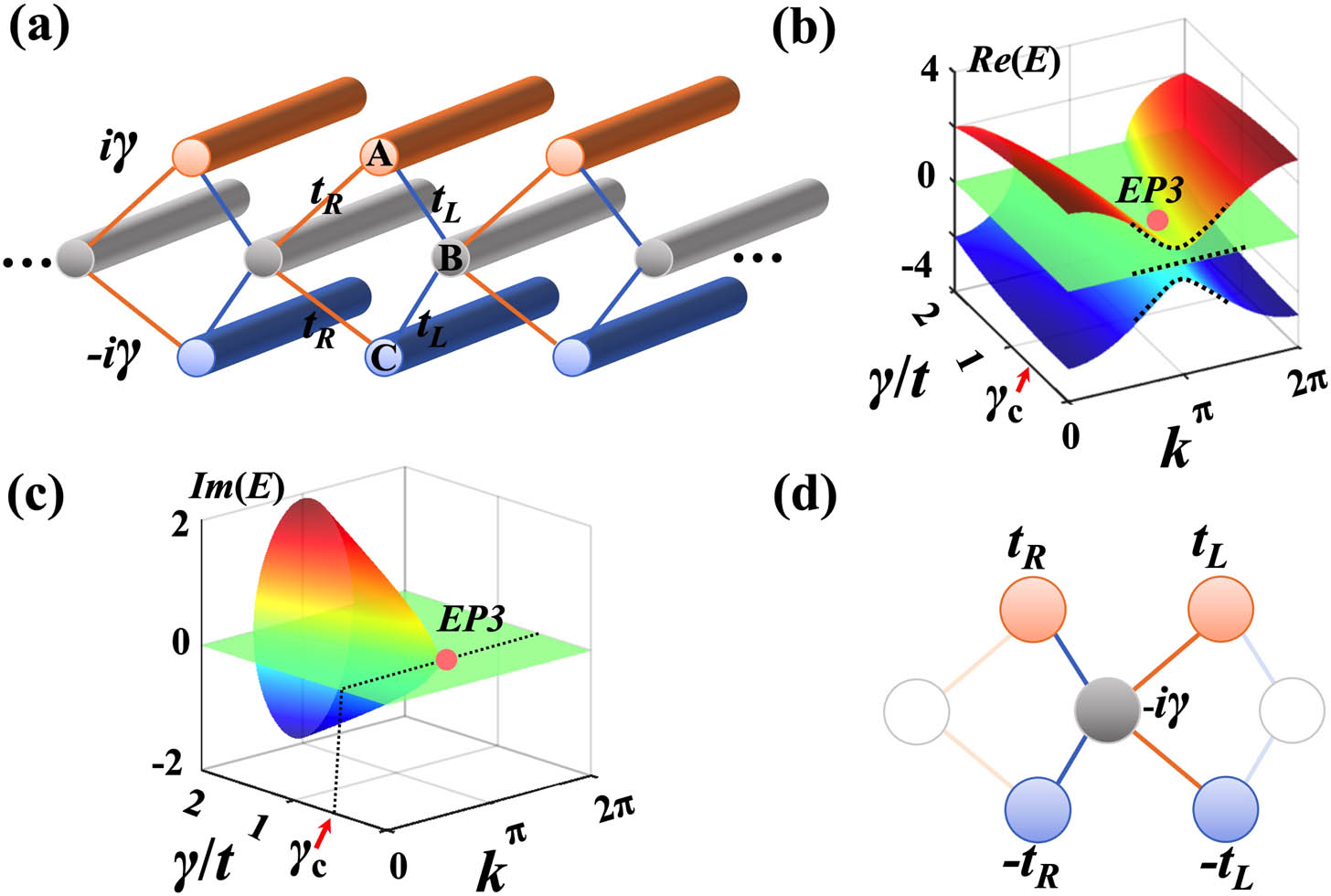
Set citation alerts for the article
Please enter your email address