Liuxin YANG, Wenhua LUO, Changan WANG, Chen XU. Novel Inorganic Two-dimensional Materials for Gas Separation Membranes [J]. Journal of Inorganic Materials, 2020, 35(9): 959

Search by keywords or author
- Journal of Inorganic Materials
- Vol. 35, Issue 9, 959 (2020)
![Schematic representation of transport mechanisms in membranes, where Dc is the location of carbon centers, and the pore diameter is defined as Dp = Dc - Dvdw/$\sqrt{2}$, where Dvdw is the van der Waals diameter of a carbon atom[1,10,11,14-17]](/richHtml/jim/2020/35/9/959/img_1.png)
1. Schematic representation of transport mechanisms in membranes, where D c is the location of carbon centers, and the pore diameter is defined as D p = D c - D vdw/$\sqrt{2}$, where D vdw is the van der Waals diameter of a carbon atom[1,10,11,14-17]
![Schematic of pores in graphene sheet (a-b) and pore electron density isosurfaces of porous graphene (c-d)[28]](/richHtml/jim/2020/35/9/959/img_2.png)
2. Schematic of pores in graphene sheet (a-b) and pore electron density isosurfaces of porous graphene (c-d)[28]
![Snapshots of passing-through events of gas molecules[29]](/Images/icon/loading.gif)
3. Snapshots of passing-through events of gas molecules[29]
![Schematic of fabrication of a large-area graphene membrane by the nanoporous carbon film-assisted transfer method (a) and high-resolution TEM images of the intrinsic defects in graphene lattice (b)[33]](/Images/icon/loading.gif)
4. Schematic of fabrication of a large-area graphene membrane by the nanoporous carbon film-assisted transfer method (a) and high-resolution TEM images of the intrinsic defects in graphene lattice (b)[33]
![Photo of a 1-µm-thick GO film (a), electron micrograph of the film’s cross section (b), schematic view for possible permeation through the laminates (c), and examples of He-leak measurements for a freestanding submicrometer-thick GO membrane and a reference PET film (d)[40] (1 mbar=100 Pa)](/Images/icon/loading.gif)
5. Photo of a 1-µm-thick GO film (a), electron micrograph of the film’s cross section (b), schematic view for possible permeation through the laminates (c), and examples of He-leak measurements for a freestanding submicrometer-thick GO membrane and a reference PET film (d)[40] (1 mbar=100 Pa)
![Schematic illustration of two different GO coating methods (Method one and Method two) (a), schematic illustration of possible gas transport through graphene membrane (b), AFM images of GO membrane surfaces with insert images showing depth profiles of GO membrane surfaces: (c) method one [root mean square roughness (Rq) = 0.8 nm, average roughness (Ra) = 0.614 nm] and (d) method two (Rq = 0.608 nm, Ra = 0.467 nm)[19]](/Images/icon/loading.gif)
6. Schematic illustration of two different GO coating methods (Method one and Method two) (a), schematic illustration of possible gas transport through graphene membrane (b), AFM images of GO membrane surfaces with insert images showing depth profiles of GO membrane surfaces: (c) method one [root mean square roughness (R q) = 0.8 nm, average roughness (R a) = 0.614 nm] and (d) method two (R q = 0.608 nm, R a = 0.467 nm)[19]
![Schematic representation of the assembly of GO nanosheets in polymeric environment (a), digital photographs of the membrane with 0.1wt% GO (b), overview (the yellow dashed lines are eye-guiding lines indicating the GO laminates in these regions) (c) and expanded TEM image of the cross section of GO-1 membrane (d)[17]](/Images/icon/loading.gif)
7. Schematic representation of the assembly of GO nanosheets in polymeric environment (a), digital photographs of the membrane with 0.1wt% GO (b), overview (the yellow dashed lines are eye-guiding lines indicating the GO laminates in these regions) (c) and expanded TEM image of the cross section of GO-1 membrane (d)[17]
![Force analysis for one 2D channel unit consisting of GO nanosheets and polymer chain (a), schematic illustration of intrinsic force induced disordered structure (left) and highly ordered laminar structures (right) driven by introduced synergistic external forces (b)[54]](/Images/icon/loading.gif)
8. Force analysis for one 2D channel unit consisting of GO nanosheets and polymer chain (a), schematic illustration of intrinsic force induced disordered structure (left) and highly ordered laminar structures (right) driven by introduced synergistic external forces (b)[54]
![Schematic illustration of the gas solution-diffusion transport pathway through a GO-SILM (a), molecular structures of [BMIM][BF4] IL confined in the GO nanochannels (b), the cross section of the GO membrane (c) and GO-SILM (d), respectively[55]](/Images/icon/loading.gif)
9. Schematic illustration of the gas solution-diffusion transport pathway through a GO-SILM (a), molecular structures of [BMIM][BF4] IL confined in the GO nanochannels (b), the cross section of the GO membrane (c) and GO-SILM (d), respectively[55]
![Schematic of gas permeation pathway across MoS2 membranes before and after heating at 160 ℃(a)[63], cross section SEM images of the membrane (b-e)[64], and synthesis process for MoS2-SILM (f)[69](b) GO membrane; (c) MoS2 membrane; (d) GO-MoS2 (50/50) hybrid membrane; (e) GO-MoS2 (75/25) hybrid membrane](/Images/icon/loading.gif)
10. Schematic of gas permeation pathway across MoS2 membranes before and after heating at 160 ℃(a)[63], cross section SEM images of the membrane (b-e)[64], and synthesis process for MoS2-SILM (f)[69](b) GO membrane; (c) MoS2 membrane; (d) GO-MoS2 (50/50) hybrid membrane; (e) GO-MoS2 (75/25) hybrid membrane
![SEM image of the delaminated MXene nanosheets on porous anodic aluminum oxide (AAO) (a) with insert showing the Tyndall scattering effect in MXene colloidal solution in water, cross-sectional SEM image of the MXene membrane (b) with insert showing a tweezer bent membrane, AFM image of the Mxene nanosheet on cleaved mica (c), illustration of the spacing between the neighboring MXene nanosheets in the membrane (d)[77], structures and gas transport of H2-selective and CO2-selective Mxene nanofilms (e)[78]](/Images/icon/loading.gif)
11. SEM image of the delaminated MXene nanosheets on porous anodic aluminum oxide (AAO) (a) with insert showing the Tyndall scattering effect in MXene colloidal solution in water, cross-sectional SEM image of the MXene membrane (b) with insert showing a tweezer bent membrane, AFM image of the Mxene nanosheet on cleaved mica (c), illustration of the spacing between the neighboring MXene nanosheets in the membrane (d)[77], structures and gas transport of H2-selective and CO2-selective Mxene nanofilms (e)[78]
|
Table 1. Graphene-based membranes for gas separation
|
Table 2. Other novel inorganic 2DMs for membrane gas separation
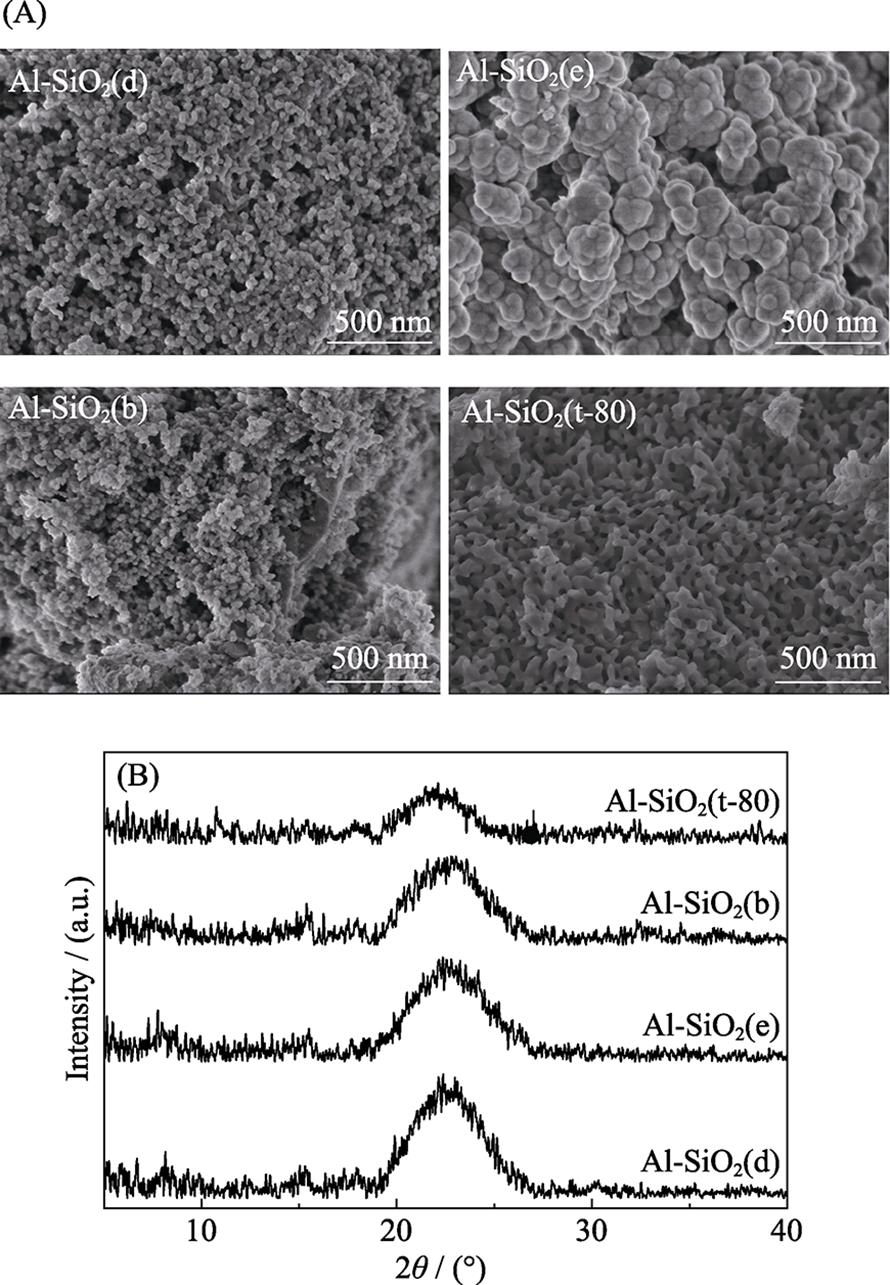
Set citation alerts for the article
Please enter your email address