Sven Ebel, Yadong Deng, Mario Hentschel, Chao Meng, Sören im Sande, Harald Giessen, Fei Ding, Sergey I. Bozhevolnyi, "Optical reflective metasurfaces based on mirror-coupled slot antennas," Adv. Photon. Nexus 2, 016005 (2023)

Search by keywords or author
- Advanced Photonics Nexus
- Vol. 2, Issue 1, 016005 (2023)
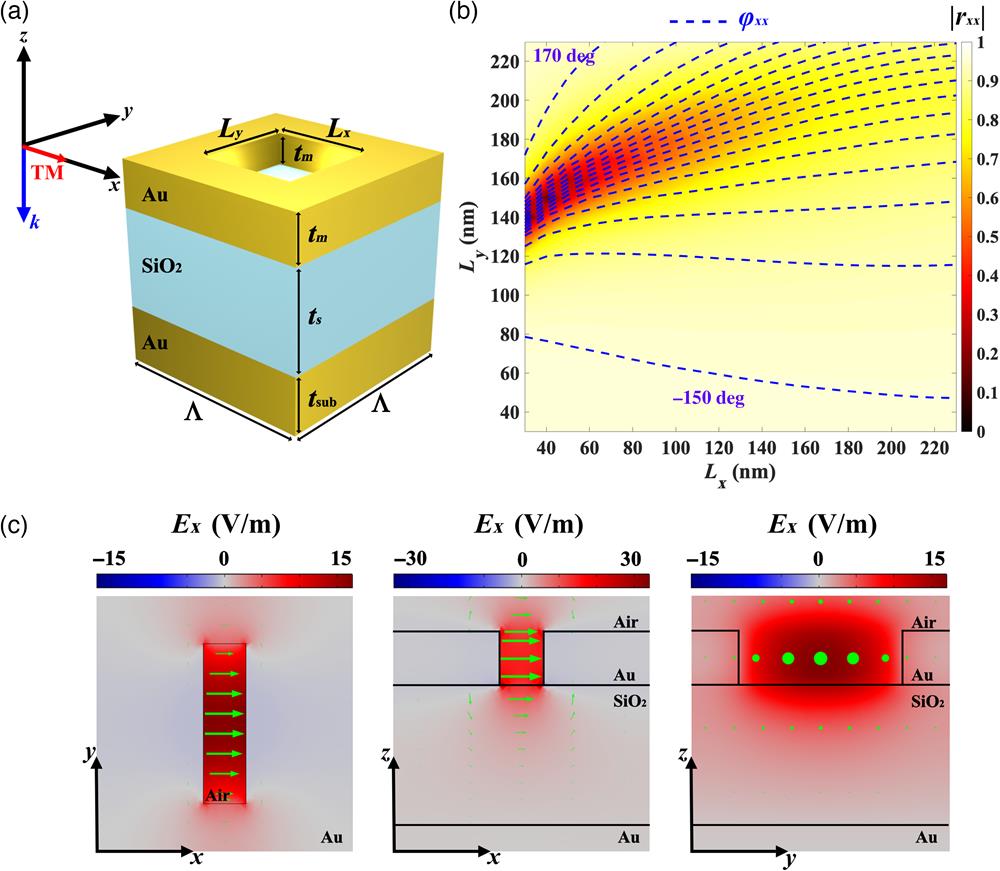
Fig. 1. (a) Schematic of the basic MCSA unit cell consisting of a slot antenna on top of an spacer and an Au mirror. (b) Numerically calculated complex reflection coefficients as a function of the slot antenna lateral dimensions at the design wavelength of upon TM polarization. The spacer thickness is set to . The amplitude is visualized by the color map, while the dashed blue lines are the contours of the reflection phase ascending by a 20-deg phase step. (c) Cross-sectional electric field distributions in different cutting planes for the slot antenna with and at . The selected cutting planes display the mode profiles within the center of the slot antenna in the top Au layer. The color bars are chosen for illustrating the mode amplitude profiles of the -component, while arrows indicate the electric field vectors at a representative moment of time.
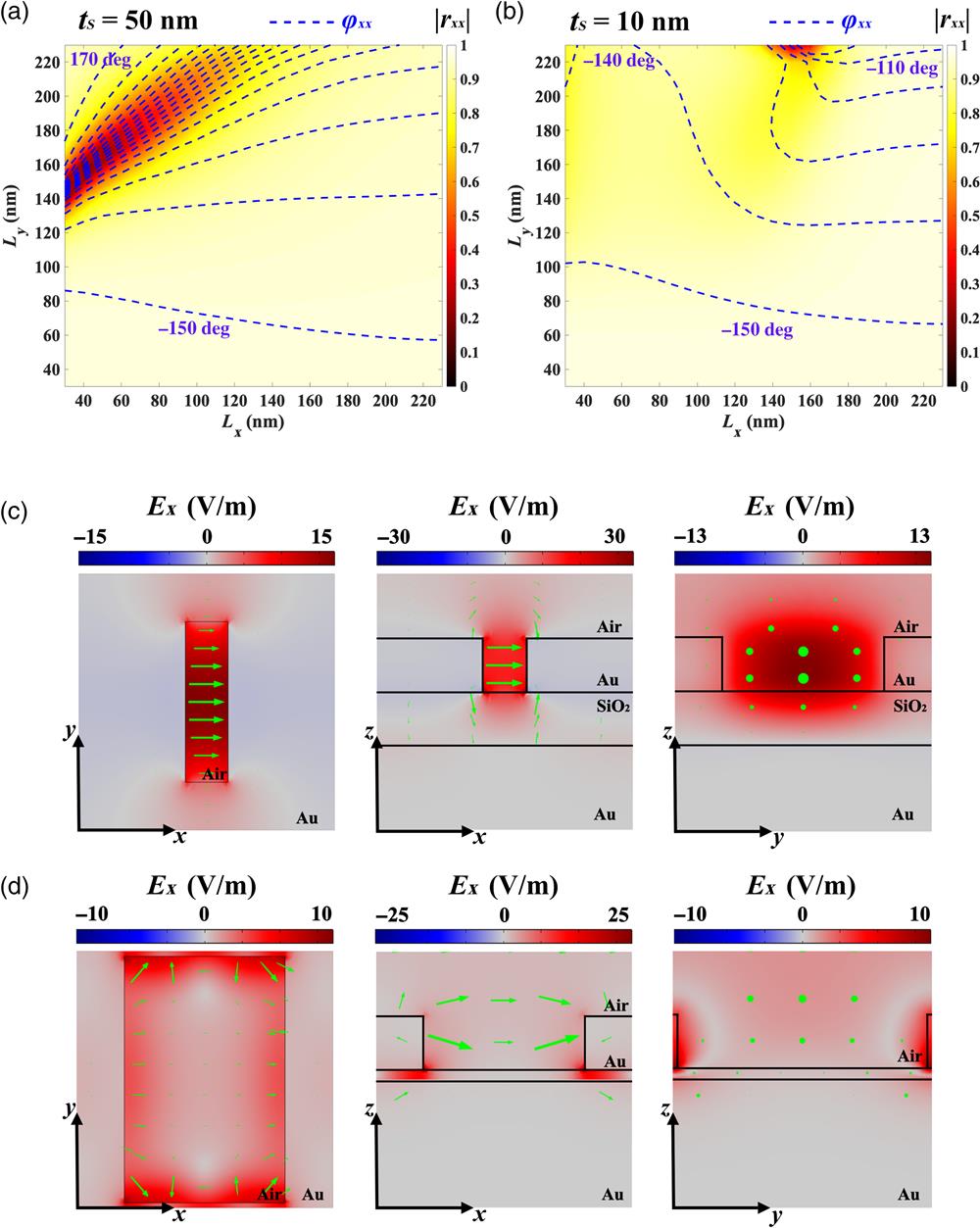
Fig. 2. (a), (b) Numerically calculated complex reflection coefficients as a function of the slot antenna’s lateral dimensions at the design wavelength of upon TM polarization. The amplitude is visualized by the color map, while the dashed blue lines are the contours of the reflection phase ascending by (a) a 20-deg phase step and (b) a 10-deg phase step. The spacer thickness is set to in (a) and in (b). (c), (d) Cross-sectional electric field distributions in different cutting planes for the slot antenna at . The selected cutting planes display the mode profiles within the center of the slot antenna in the top Au layer. The color bars are chosen for illustrating the mode amplitude profiles of the -component, while arrows indicate the electric field vectors at a representative moment of time. (c) The spacer thickness is set to and the slot-antenna dimensions are and . (d) The spacer thickness is set to and the slot-antenna dimensions are and .
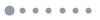
Fig. 3. Theoretical performance of the beam-steering MCSA metasurfaces that reflect the normal incident TM-polarized light into the +1 diffraction order for supercells consisting of (a) pair, (b) triple, and (c) quadruple identical MCSA meta-atoms. The left columns display the simulated diffraction efficiencies of different orders as a function of wavelength. The right columns show the electrical field distributions at the plane cut from the center of the top slot-antenna layer at the wavelength of .
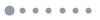
Fig. 4. (a) Schematic illustration of the MCSA reflective metasurface for beam steering. (b) SEM images of the fabricated beam-steering metasurface. The lower image depicts the fabricated supercells. (c) Simulated (solid lines) and experimental (dots with error bars) diffraction efficiencies of different orders as a function of wavelength for TM incident light. The error bars denote the standard deviation of the measured data of four metasurface samples. The inset image shows the diffracted light spots at , whose intensity has been adjusted for the visualization.
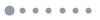
Fig. 5. (a) Schematic illustration of the MCSA reflective metasurface for beam splitting. (b) SEM images of the fabricated beam-splitting metasurface. The lower image shows the designed and fabricated supercells. (c) Simulated (solid lines) and experimental (dots with error bars) diffraction efficiencies of different orders as a function of wavelength for TM incident light. The error bars denote the standard deviation of the measured data of four metasurface samples. The inset image shows the diffracted light spots at , whose intensity has been adjusted for the visualization.
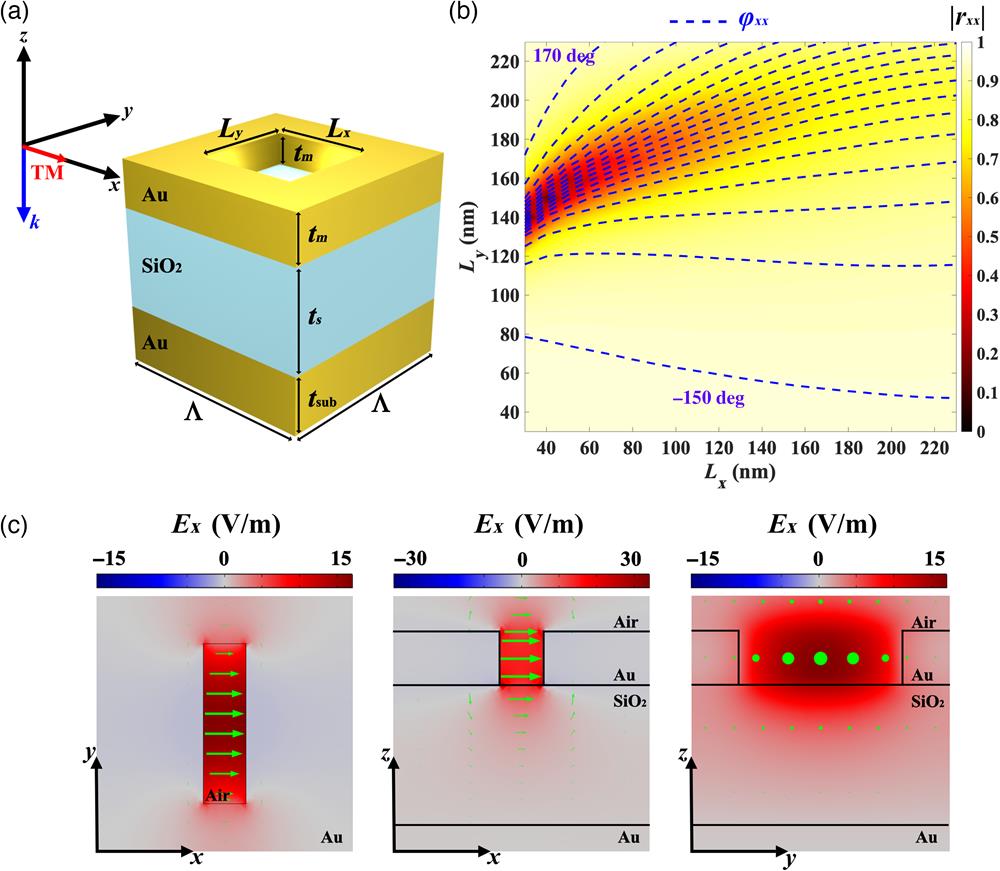
Set citation alerts for the article
Please enter your email address