Haoyang Zhou, Sheng Zhang, Shunjia Wang, Yao Yao, Qingnan Cai, Jing Lin, Xiaoying Zheng, Zhuo Wang, Zhensheng Tao, Qiong He, Lei Zhou, "Optically controlled dielectric metasurfaces for dynamic dual-mode modulation on terahertz waves," Adv. Photon. 5, 026005 (2023)

Search by keywords or author
- Advanced Photonics
- Vol. 5, Issue 2, 026005 (2023)
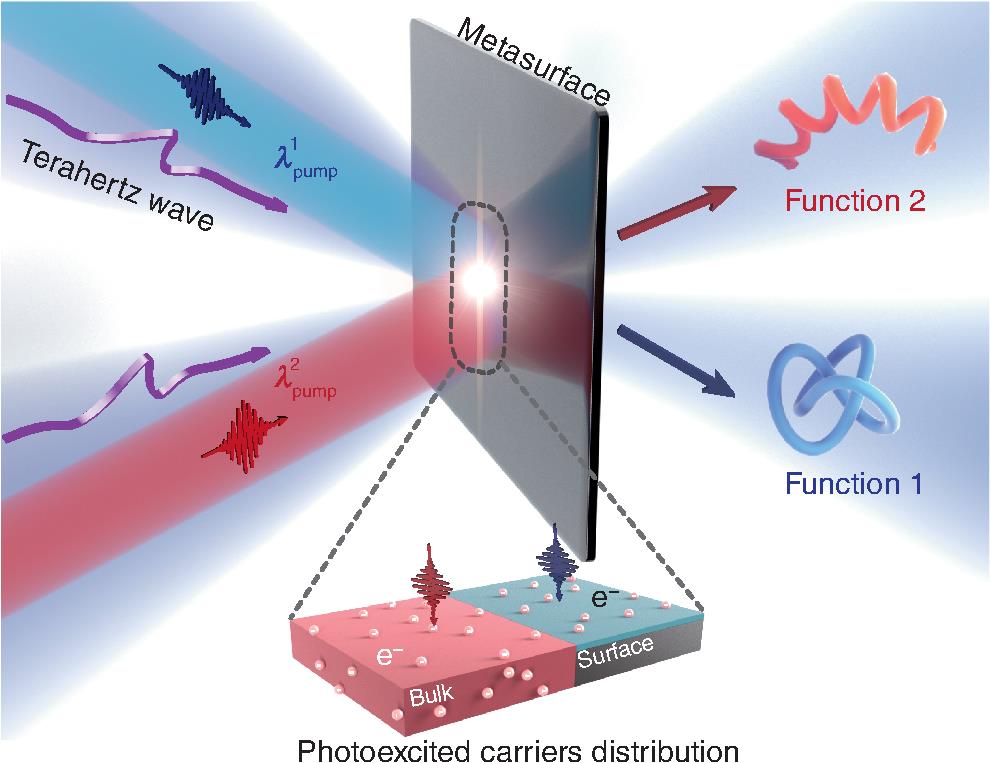
Fig. 1. Schematic of the dynamic dual-mode modulation with an optically controlled dielectric metasurface. Under pumping by external light at different wavelengths, a predesigned dielectric metasurface can exhibit distinct functionalities to manipulate THz waves.
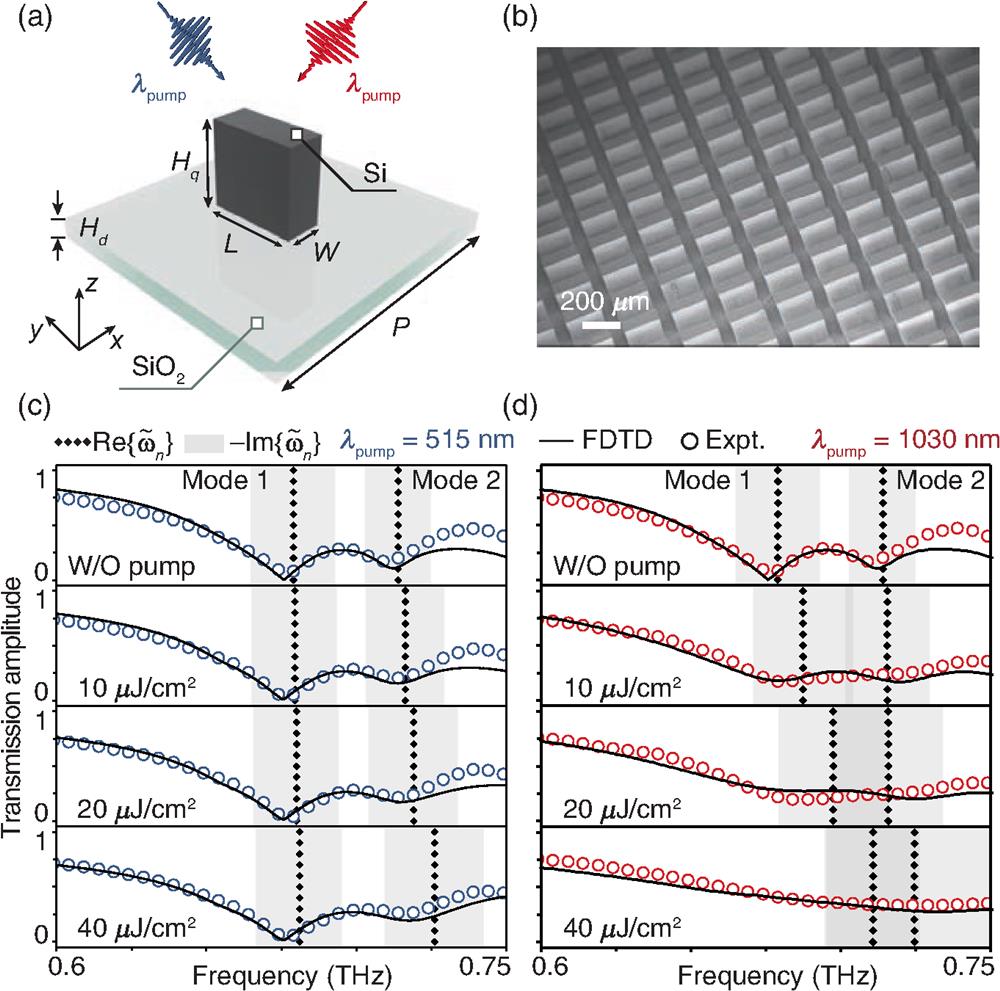
Fig. 2. Experimental demonstration of the dynamic dual-mode THz wave modulation. (a) Schematic of the basic meta-atom consisting of a quartz substrate and silicon pillar with the following geometrical parameters: , , , , , all in units of . (b) Tilt-view SEM image of the fabricated sample. (c), (d) Measured (open circles) and simulated (solid lines) transmission amplitude spectra of the fabricated metasurface, under external pumping at (c) 515 nm and (d) 1030 nm with different pump fluences. Black dotted vertical lines and gray shaded areas denote the real part and negative imaginary part of calculated by the QNM theory in different cases.
![Working mechanism of the dynamic dual-mode modulation. (a) The solid line represents the calculated penetration depth of silicon versus the wavelength; the blue star and red triangle represent the penetration depths of silicon for pump light at 515 and 1030 nm, respectively; the left (right) inset depicts the perturbed region [highlighted by red (blue) color] inside the silicon pillar pumped by external light at 515 nm (1030 nm). (b) FEM-simulated normalized spatial distributions of |E˜·E˜| at eigen frequencies of Modes 1 and 2 inside the silicon resonator. (c)–(f) Numerically simulated and analytically calculated values of (c), (d) −Im(Δω˜n) and (e), (f) Re(Δω˜n) for two resonant modes as varying optical fluence F of pump light at the wavelength of (c), (e) 515 nm and (d), (f) 1030 nm, respectively.](/Images/icon/loading.gif)
Fig. 3. Working mechanism of the dynamic dual-mode modulation. (a) The solid line represents the calculated penetration depth of silicon versus the wavelength; the blue star and red triangle represent the penetration depths of silicon for pump light at 515 and 1030 nm, respectively; the left (right) inset depicts the perturbed region [highlighted by red (blue) color] inside the silicon pillar pumped by external light at 515 nm (1030 nm). (b) FEM-simulated normalized spatial distributions of at eigen frequencies of Modes 1 and 2 inside the silicon resonator. (c)–(f) Numerically simulated and analytically calculated values of (c), (d) and (e), (f) for two resonant modes as varying optical fluence of pump light at the wavelength of (c), (e) 515 nm and (d), (f) 1030 nm, respectively.
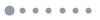
Fig. 4. Experimental demonstration of a dynamic dual-mode metapolarizer. (a) Schematic of the metapolarizer exhibiting different polarization-manipulation functionality as pumped by external light at different wavelengths. The inset depicts part of the SEM image of the fabricated sample with a scale bar (white line) of . (b) FDTD-simulated polarization trajectories on Poincaré’s sphere of THz waves at 0.695 THz passing through the metadevice pumped by external light at different wavelengths with varying pump fluences. (c) Measured (stars) and simulated (line) polarization states of THz waves at 0.695 THz transmitted through the metadevice under the photoexcitation of 515-nm (blue) and 1030-nm (red) pump light with different pump fluences. (d) Measured and simulated spectra of transmission amplitudes ( and ) and cross-polarization phase difference ( ) of the metadevice without photoexcitation. (e), (f) Measured and simulated evolutions of transmission amplitude and cross-polarization phase difference of the metadevice at 0.695 THz as a function of pump fluence under photoexcitation at the wavelength of (e) 515 nm and (f) 1030 nm, respectively.
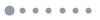
Fig. 5. Numerical demonstration of an optical information encryption metasurface. (a) Schematic of the proposed metadevice composed of two basic meta-atoms (see insets) arranged in a predesigned sequence. Geometrical parameters of meta-atom A are set as , , , , all in units of micrometers, while the length and width of meta-atom B are adjusted to and , respectively. (b) FDTD-simulated spectra of reflection amplitude and phase of two periodic metasurfaces consisting of arrays of meta-atom A (red and blue solid lines) and meta-atom B (red and blue dotted dashed lines), respectively. Gray dashed line denotes the working frequency 0.63 THz. (c) Reflection phases of two meta-atoms under different pump fluences at different wavelengths, obtained by FDTD simulations at 0.63 THz. (d)–(f) Computed normalized field distributions on the plane at above the metasurface shone by normally incident THz wave at 0.63 THz, under different pumping conditions (d) without any photoexcitation, (e) pumped by 515-nm light with fluence , and (f) pumped by 1030-nm light with fluence .
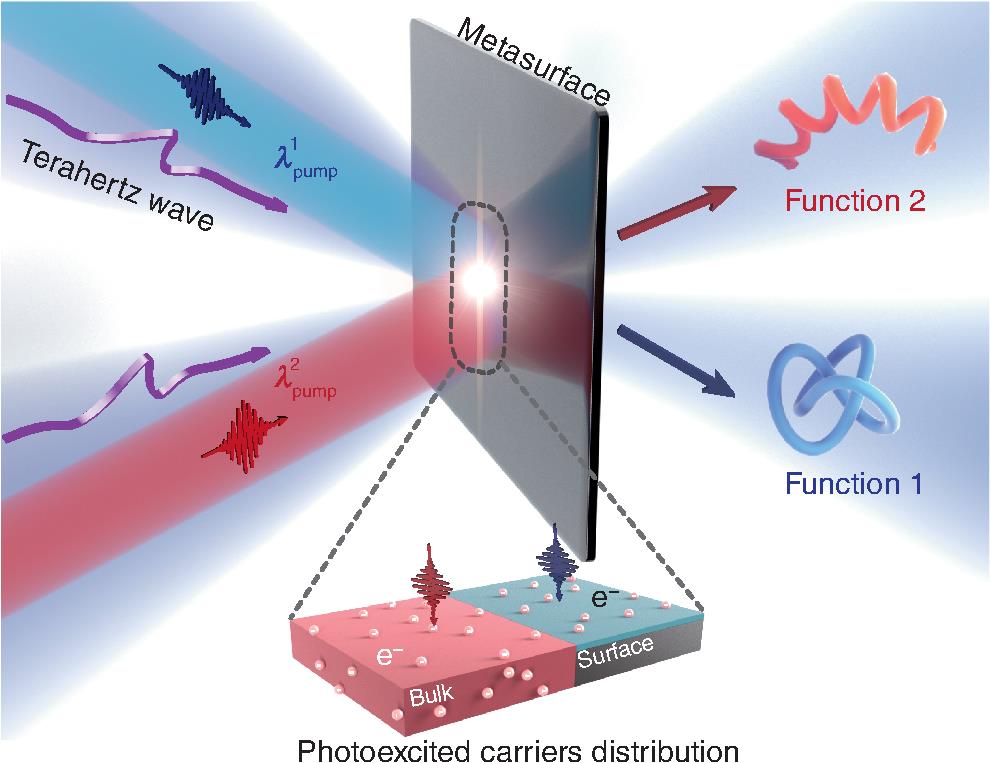
Set citation alerts for the article
Please enter your email address