Yang Li, Haolin Chen, Yanxian Guo, Kangkang Wang, Yue Zhang, Peilin Lan, Jinhao Guo, Wen Zhang, Huiqing Zhong, Zhouyi Guo, Zhengfei Zhuang, Zhiming Liu, "Lamellar hafnium ditelluride as an ultrasensitive surface-enhanced Raman scattering platform for label-free detection of uric acid," Photonics Res. 9, 1039 (2021)

Search by keywords or author
- Photonics Research
- Vol. 9, Issue 6, 1039 (2021)
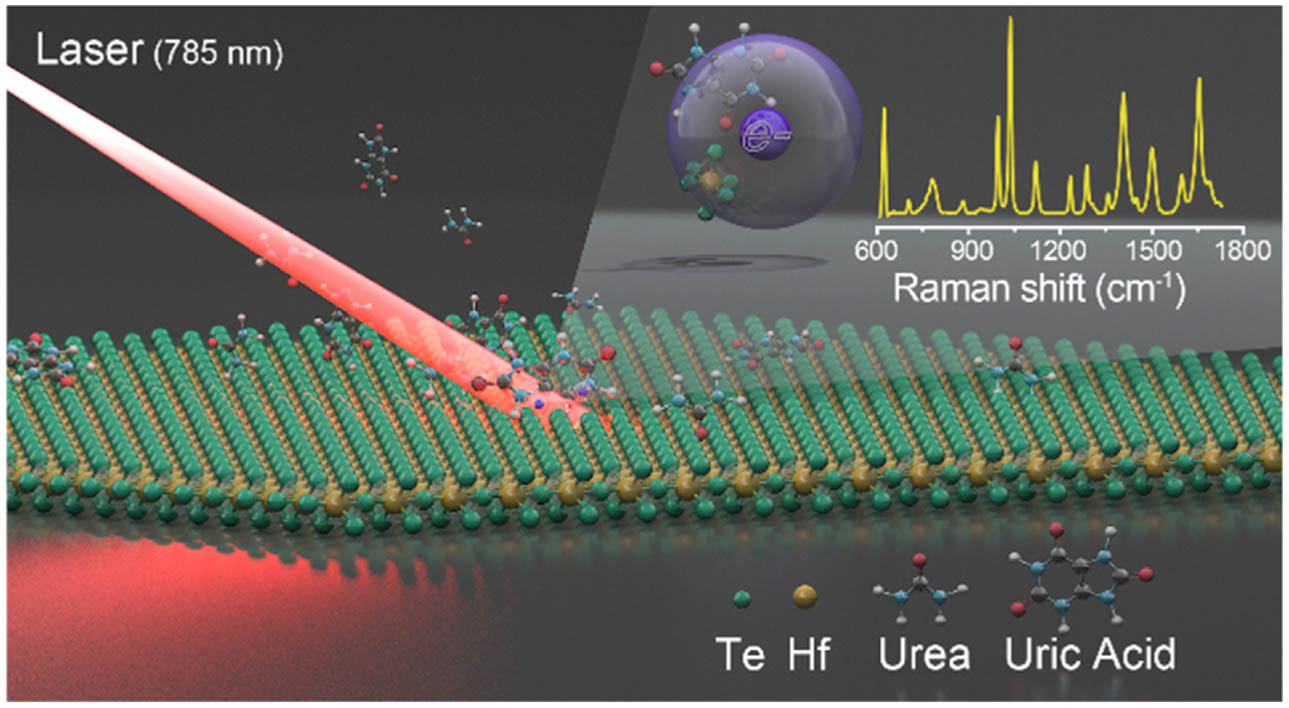
Fig. 1. Schematic illustration of the SERS detection of uric acid based on HfTe 2 nanosheets.
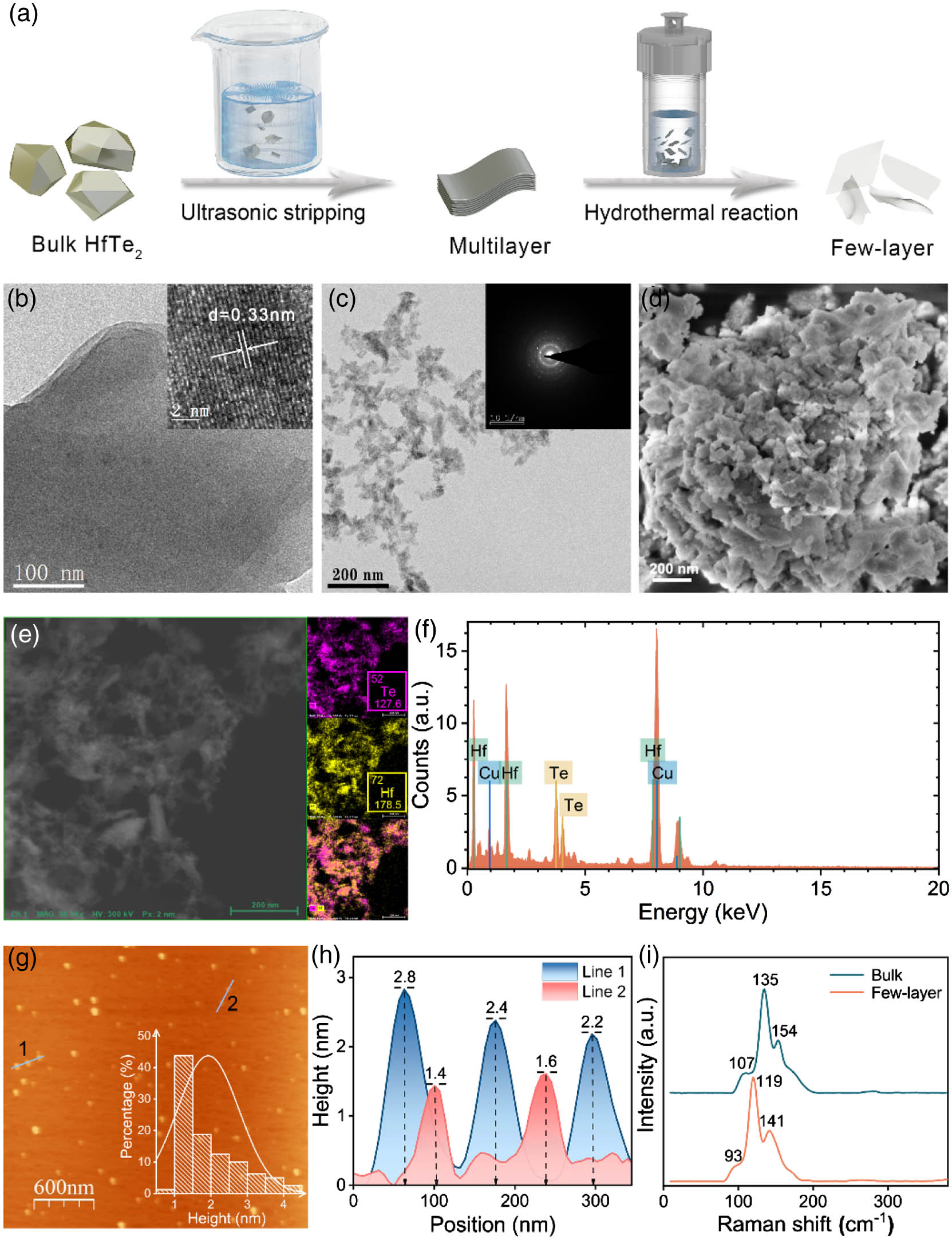
Fig. 2. Synthesis and characterization of HfTe 2 nanosheets. (a) Schematic representation of the preparation process of HfTe 2 nanosheets. (b)–(c) TEM images of multilayer and few-layered hafnium ditelluride nanomaterials. The inset shows the HRTEM image and SAED pattern, respectively. (d) SEM image of HfTe 2 nanosheets. (e) HAADF image and corresponding elemental mapping (Te and Hf) of HfTe 2 nanosheets. (f) EDX pattern of HfTe 2 nanosheets. (g) AFM image with the corresponding size distribution (inset) and (h) height analysis of HfTe 2 nanosheets. (i) Raman spectra of bulk and few-layered HfTe 2 .
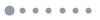
Fig. 3. Atomic structure of monolayer HfTe 2 nanosheet. (a) Left and (b) top views of lattice structures. (c) Unit cell structure of the nanostructures. Green spheres, tellurium atoms; and yellow spheres, hafnium atoms. (d) XRD pattern of HfTe 2 nanosheets.
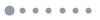
Fig. 4. (a) XPS analysis of HfTe 2 nanosheets. The high resolution XPS spectra of Hf 4f in (b) bulk and (c) few-layered HfTe 2 sheets. (d) UV-Vis-NIR absorbance spectrum. (e) Typical optical absorption curve of HfTe 2 nanosheets, where E g is estimated to be 4.93 eV. (f) Valence band spectrum of HfTe 2 nanosheets, where E vb is calculated to be − 4.98 eV .
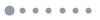
Fig. 5. (a) Raw Raman spectra of Rh6G (10 − 3 M ) dye on Si wafer with or without HfTe 2 substrate. (b) SERS spectra of different concentrations of Rh6G on HfTe 2 substrate. (c) Intensity values of typical Raman peaks in (b). Fitting curve of the inset is the SERS intensity-concentration plot for 1313 cm − 1 (R 2 ) band of Rh6G. (d) EF values of five typical Raman peaks of Rh6G at different concentration levels.
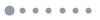
Fig. 6. (a) Cluster of SERS spectra of Rh6G samples randomly collected at 20 sites on the HfTe 2 substrate. (b)–(d) SERS intensities of three typical peaks at 1514 cm − 1 , 1365 cm − 1 , and 1313 cm − 1 in the 20 SERS spectra.
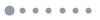
Fig. 7. (a) SERS image of Rh6G molecules (1514 cm − 1 ) on HfTe 2 nanosheets. (b) Contour map of 170 SERS spectra collected from the Raman mapping. (c) Average spectrum (blue line) of the 170 spectral data and the reconstructed spectrum (green line) along the green diagonal line in (b).
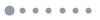
Fig. 8. Schematic diagram of the photo-induced charge transfer process between HfTe 2 and Rh6G under 785 nm laser excitation.
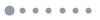
Fig. 9. Mean SERS spectra of pure uric acid, urea, and their mixture on HfTe 2 nanosheets.
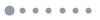
Fig. 10. (a) SERS detection of UA at different concentrations on HfTe 2 nanosheets. Curves a to j: SERS spectra of UA at 1000, 600, 500, 400, 350, 300, 250, 200, 150, and 100 μM, respectively. Curve k: normal Raman spectrum of UA at 1 mM. (b) Fitting curve of SERS intensity-logarithmic concentration for 1039 cm − 1 band of UA. (c) SERS spectra of UA (0.1–0.5 mM) on HfTe 2 nanosheets in the presence of 4 mM urea. (d) Calibration curve by plotting the peak intensity ratio (I 1039 / I 1012 ) as a function of UA concentration. Enlargements of the typical Raman peaks (urea 1012 cm − 1 , uric acid 1039 cm − 1 ) are shown as insets.
|
Table 1. Calculated Maximum EFs of Typical SERS Peaks of Different Dyes on Substrate
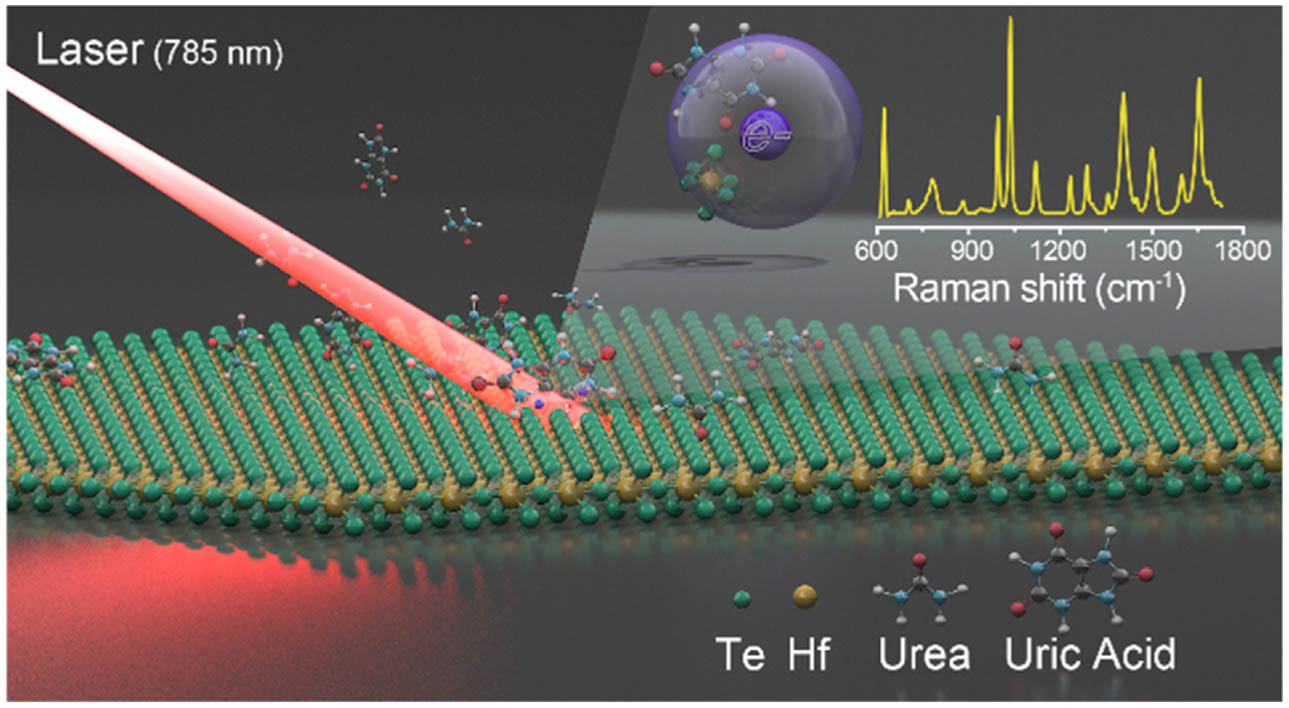
Set citation alerts for the article
Please enter your email address