
- Journal of Inorganic Materials
- Vol. 35, Issue 10, 1169 (2020)
Abstract
Natural enzyme is produced by living cells, and participates in almost all life activities, which has a strong specific catalytic activity and a well application prospect in many fields[
Ascorbic acid is an essential nutrient for the human body. It has functions of regulating human immunity, preventing gum bleeding and promoting certain amino acid metabolism[
Therefore, we adoped a facile modified polyol process, using polyvinylpyrrolidone (PVP) as a surface blocking agent, a certain concentration of hydrochloric acid as an oxidizing etchant, and prepared Pt-Au dendritic nanoparticles by adjusting precursor ratio and reaction temperature. The oxidase-like activity was tested using TMB as a chromogenic substrate. Furthermore, we have established a new method for colorimetric detection of ascorbic acid, in which the presence of AA can cause a change in the color of the reaction system and is visible.
1 Experimental
1.1 Chemicals
Chloroplatinic acid hexahydrate (H2PtCl6·6H2O, chloroauric acid trihydrate (HAuCl4∙3H2O) were obtained from Sigma-Aldrich. And ethylene glycol (C2H6O2), 3,3′,5,5′-tetramethylbenzidine (TMB), PVP were purchased from Alfa Aesar. Hydrochloric acid, sulfuric acid, ethanol, n-hexane, and acetic acid- sodium acetate buffer solution (0.2 mol/L, pH 4.0) were purchased from Aladdin, China. The water used was deionized water (18 MΩ·cm). All the chemicals were used without further purification.
1.2 Characterization
Absorbance spectra of the reaction solution was measured using an ultraviolet-visible spectrophotometer (Lamda950), and the cuvette path length was 1 cm. A transmission electron microscope (TEM, Tecnai G2 F20) and a scanning electron microscope (SEM, FEI-Magellan) were used to observe the sample morphology. The infrared spectrum was obtained by a Fourier infrared spectrometer (NICOLET Is10). X-ray diffraction (XRD) characterization was performed by a D8 ADVANCE diffractometer.
1.3 Synthesis of Pt-Au dendritic nanoparticles
In a 50 mL three-necked flask, 2.5 mL of ethylene glycol was refluxed at 180 ℃ for 5 min with oil bath. After which, 0.34 mL of HCl (0wt%-37wt%) was added. Then, 0.94 mL of a mixed solution of H2PtCl6·6H2O (0.0625 mol/L) and HAuCl4∙3H2O (0.0625 mol/L) (both in molor ratio of 9 : 1-5 : 1) and 4 mL of PVP were added into the above solution for 10 times within 5 min. During the reaction, the reactants were continuously stirred and mixed uniformly. After 20 min, the flask was taken out, naturally cooled to room temperature. Finally, the sample was collected by centrifugation.
1.4 Kinetic assay of oxidase-like Pt-Au DNPs
60 μL Pt-Au DNPs (0.75 mg/mL), 140 μL of TMB, were added to 2.8 mL of acetic acid-acetate buffer solution (0.2 mol/L, pH 4.0) at 35 ℃. The kinetic measurements were performed in a time course mode using a spectrophotometer to measure the absorbance spectra at the first 3 min of the reaction system at 652 nm every 15 s. Lineweaver-Burk Graphs was used to calculate Michaelis-Menten constants (Km):
1/v=Km/Vmax(1/[S]+1/Km)
where [S] is the substrate concentration, and Vmax is the maximum velocity of the reaction. Km: Michaelis constant, Vmax: maximum reaction rate.
1.5 Oxidase-like activity of Pt-Au DNPs
40 μL TMB (5 mmol/L) and 60 μL Pt-Au DNPs solution (0.75 mg/mL) were added to 2.9 mL acetic acid- sodium acetate buffer solution (0.2 mol/L, pH 4.0), then incubated the solution at 35 ℃ with water bath. UV- visible absorption spectra were used to trace the reaction.
1.6 Detection of ascorbic acid (AA)
40 μL TMB(5 mmol/L) and 60 μL Pt-Au DNPs solution (0.75 mg/mL) were added to 2.8 mL acetic acid- sodium acetate buffer solution (0.2 mol/L, pH 4.0). After that, the mixture was incubated at 35 ℃ for 10 min. Than 50 μL AA solution were added into it. Their UV- visible absorption spectra were measured at 200-800 nm.
2 Results and discussion
2.1 Characterization of Pt-Au dendritic nanoparticles
Fig. 1(a, b) show SEM and TEM images of the synthesized Pt-Au DNPs, with diameter of about 35 nm. It can be clearly seen that the nanoparticles have a distinct dendritic structure, which has the effect of increasing the specific surface area of the prepared nanomaterial. At the same time, the agglomeration of nanoparticles is a little serious, probably due to the large specific surface area (Fig. 1(a)). The HRTEM further indicates that the lattice spacing of the parallel stripes is 0.231 nm (Fig. 1(c)), which corresponds to the (111) crystal plane and between the lattice constant of Pt (0.228 nm) and Au (0.235 nm)[
Figure 1.(a) SEM and (b) TEM images of the as-synthesized Pt-Au DNPs (after 20 min reaction); (c) HRTEM image, (d) STEM image and EDS mapping for Au, Pt elements distribution of one single Pt-Au nanoparticle (d-1: Au and d-2:Pt)
The reaction time is critical to the morphology of as-synthesized Pt-Au DNPs. To explore the formation process, the nanoparticles prepared for different reaction-time were characterized. As show in Fig. 2, the nanoparticles which had dendritic structure were observed after the reaction for 5 min (Fig. 2(a)). When the reaction time was 10 min, the dendritic particles grew bigger (Fig. 2(b)). Until the reaction was extended to 20 min (Fig. 2(c)), Pt-Au DNPs were obtained with good dispersibility, high dendritic structure and uniform size. The yielded nanoparticles became smaller and the dendritic structure gradually disappeared when the reaction time was 40 min (Fig. 2(d)), which may result from the fact that the prolonged heat treatment damaged the instable nanoparticle structure. This indicates that reasonable control of reaction time is helpful to obtain materials with good morphology and excellent properties.
Figure 2.TEM images of Pt-Au DNPs prepared for at (a) 5, (b) 10, (c) 20, and (d) 40 min
It is known from the existing literature that HCl is an important additive for the formation of dendritic structure, and O2/Cl- is a commonly used oxidizing etchant and has the function of regulating the morphology of nanoparticles[
Figure 3.TEM images of Pt-Au DNPs obtained by adding different concentrations of HCl (a) 0wt%; (b) 18.5wt%; (c) 37%wt%
PVP is also a commonly used morphology control agent for the preparation of noble metal nanoparticles, which can be adsorbed on the crystal surface to regulate anisotropic growth. At the same time, PVP acts as a stabilizer and a blocking agent, and also could protect the nanoparticles from agglomeration[
Figure 4.TEM images of Pt-Au DNPs obtained with different concentrations of PVP (a) 0.0187 mol/L; (b) 0.0375 mol/L; (c) 0.0750 mol/L
2.2 Oxidase-like activity of Pt-Au dendritic nanoparticles
Using TMB as a substrate, the oxidase-like activity of Pt-Au DNPs was assessed by testing the degree of oxidation of TMB without adding H2O2. As shown in Fig. 5, Pt-Au DNPs catalyzed the oxidation of TMB to produce a typical blue product with a strong absorption peak at 652 nm, which is consistent with the literature [
Figure 5.UV-visible absorption spectra of different systems
2.3 Optimization of different experimental conditions
Different experimental conditions had a great influence on the oxidase-like activity of Pt-Au DNPs. Therefore, the optimal reaction conditions were explored by changing the concentration of Pt-Au DNPs, temperature, pH, and reaction time. As shown in Fig. 6(b, d), the absorption intensity of the reaction system reached the maximum at 652 nm when the temperature was lower at 35 ℃ and the pH of the reaction solution was 4.0, indicating that the activity of Pt-Au DNPs was strongest under this reaction conditions. The above results could be confirmed by the color depth of ox-TMB solution (inset in Fig. 6(c, d)). Similarly, the TMB oxidation reaction under different concentrations of catalyst (Fig. 6(a, c)) showed that the absorption intensity increased with the increase of Pt-Au DNPs concentration and reaction time. When the concentration of Pt-Au DNPs reached 15 μg/mL and the reaction time reached about 8 min, the increasing rate of absorption intensity gradually slowed down. Therefore, to facilitate experimentation and save catalyst usage, the following experiments were carried out using the reaction conditions of pH 4.0, temperature 35 ℃, 15 μg/mL Pt-Au DNPs, and 10 min of reaction time.
Figure 6.Effects of different experimental conditions on the oxidase-like activity of Pt-Au DNPs. The Absorbance spectra and visual color changes of TMB in presence of different (a) Pt-Au DNPs concentration, (b) pH, (c) time, (d) temperature, respectively, with insets showing the corresponding photos of the reaction solutions
2.4 Steady-state kinetic assay of Pt-Au DNPs
To further understand oxidase activity of Pt-Au DNPs, the time course mode was used to explore initial velocity of the reaction and kinetic parameters. The Michaelis-Menten curve was established by changing TMB concentration and tracking the absorbance of the reaction solution at 652 nm for the first 3 min of the reaction (Fig. 7(a)). At the same time, the Km of Pt-Au DNPs for TMB oxidation was calculated to be 0.22 mmol/L, and the Vmax was 282 nmol/(L·s) according to the Lineweaver- Burk plot (Fig. 7(b)). For natural enzymes, the smaller the Km, the higher the affinity of the enzyme to the substrate. At the same time, as shown in Table S2, the Km obtained in this experiment was compared with those reported in other literatures, and the value was found to be small, indicating that the affinity of Pt-Au DNPs with TMB is high. Comparing the measured Km with the products obtained by changing the reaction parameters, it’s found that the lower Km value was accompanied by a better morphology of the catalyst (obvious and dispersioned dendritic structure). But when preparing the product by doubling PVP amount, the Km value was rather high (Fig. S7), which might link to the PVP adsorption on the surface of the nanoparticles occupying the active site.
Figure 7.(a) Michaelis-Menten curve and (b) Lineweaver-Burk plots of Pt-Au DNPs with TMB concentration ([TMB])
2.5 Inhibition of ascorbic acid on oxidase-like activity of Pt-Au DNPs
To better understand the catalytic process, different concentrations of AA were added before the reaction, and then the intensity of absorbance peak at 652 nm was measured as a function of time. Fig. 8(a) showed that AA delayed the reaction, and the higher the AA concentration, the stronger the retardation was, but there were not substantially effect on the plot slope. It indicated that AA was an effective antioxidant, and TMB started to oxidize when AA was consumed. As shown in Fig. 8(b), the absorption intensity decreased instantaneously when AA was added, indicating that AA could effectively reduce the already oxidized TMB, and over time, the reduced TMB was oxidized again. However, as the number of AA additions increased, the TMB oxidation rate became slower. This implied that the continuous reaction would reduce the catalytic performance of Pt-Au DNPs, which might be related to catalyst being poisoned. Meanwhile, the performance of Pt-Au DNPs was severely reduced by continuous reaction (Fig. S8(a)), but centrifugal washing could obviously restore its performance. Fig. S8(b) showed that after prolonging the reaction time to re-oxidize TMB to the same level as the first test, the limit of detection (LOD) (87 nmol/L) obtained by retesting AA was not much different from the first test (78 nmol/L), indicating the possibility of recycling. At the same time, Pt-Au DNPs could directly oxidize AA (Fig. S9). Based on the above research, the scheme and design principle of testing AA are shown in Scheme 1, containing two steps: TMB is oxidized to ox-TMB, and ox-TMB is reduced by AA.
Figure 8.Time-dependent absorbance for (a) Pt-Au DNPs catalyzed TMB oxidation in the presence of different concentrations of AA and (b) ox-TMB reduced by AA
2.6 Detection of ascorbic acid
As described above, Pt-Au DNPs have oxidase-like activity and can catalyze the oxidation of TMB to form a blue product (ox-TMB). By using AA to inhibit the activity of Pt-Au DNPs and reduce ox-TMB, a new colorimetric assay was formed. Based on this detection method described in above Materials and Methods, a standard curve for detecting AA was established. As shown in Fig. 9(a), the absorbance value at 652 nm gradually decreased with increasing AA concentration, indicating that the content of ox-TMB and the addition of AA content satisfied a certain relationship. The analytical curve showed a linear range of 1-15 μmol/L (Fig. 9(a)). After calculation, the LOD was 78 nmol/L. It indicated that our method was more sensitive than those previously reported results (Table S3). Fig. S10 showed that the product performance was best when the precursor ratio is 5 : 1. To test the selectivity of this method, several ions (HCO3-, Na+, Br-, K+, Al3+, NO3-, Cu2+, Cl-, I-, F-, SO42-, Zn2+, CH3COO-), glucose, lysine, and so on were used. However, the results showed that these substances had little effect on the reduction of oxidase-like activity of ox-TMB and Pt-Au DNPs, which could be neglected (Fig. 9(b)). After the method was put into detecting AA in real sample (Table S1), the recoveries of the sample range were from 103.68% to 103.71%. Therefore, the method is considered to be an effective tool for determining AA content.
Figure 9.(a) Dose-response curve for different concentrations of AA standard solutions, with inset showing the linear calibration plot for AA, and (b) interference of different interfering substances in the AA detection, all interfering substances were at a concentration of 25 μmol/L
3 Conclusions
In summary, Pt-Au DNPs is synthesized through a modified polyol process, in which PVP and HCl are served as shape-control agent and the oxidase-like activity of nanoparticles can be adjusted by controlling the amount of the additions. Kinetic studies show a typical Michaelis-Menten kinetics, indicating that Pt-Au DNPs has good affinity for TMB. And AA can effectively inhibit the oxidation of TMB, while reducing the already ox-TMB, and AA itself can also be oxidized by Pt-Au DNPs. In particular, Pt-Au DNPs has a certain reusable potential. Based on above reaction process, a simple colorimetric method is established for detecting AA with high sensitivity and selectivity. This study provides some ideas for its application in food and drug testing, optical sensing and other aspects.
Supporting materials
Supporting materials related to this article can be found at
CHENG Qin1,2, YANG Yong2, YANG Lili2
1. Shanghai Applied Radiation Institute, Shanghai University, Shanghai 201800, China; 2. State Key Laboratory of High Performance Ceramics and Superfine Microstructure, Shanghai Institute of Ceramics, Chinese Academy of Sciences, Shanghai 200050, China
Figure S1.EDS elemental analysis of Pt-Au DNPs
Figure S2.XRD pattern Pt-Au DNPs
Figure S3.Color change of the solution with different concentration of HCl after reaction for 5 min
Figure S4.FT-IR spectra of Pt-Au DNPs
Figure S5.TMB oxidation catalyzed by different NPs
Figure S6.TMB oxidation catalyzed by Pt-Au NPs with different Au/Pt ratio
Figure S7.Effect of preparation conditions on values of Michaelis constant for TMB oxidation
Figure S8.(a) Pt-Au DNPs continuously catalyzed the oxidation of TMB (each time incubation for 10 min after reducing ox-TMB by AA, with the fourth time showing the result of centrifugal washing of Pt-Au DNPs before incubation), and (b) linear calibration curve for AA after 20 min of TMB second oxidation
Figure S9.Evolutions of the absorption of AA at 264 nm over time with and without Pt-Au DNPs
Figure S10.Linear calibration plot for AA of Pt-Au NPs
Sample | Added/(μmol·L-1) | Found/(μmol·L-1) | Recovery/% | RSD/(%, |
---|---|---|---|---|
Juice | 0 | 5.53 | - | 4.1 |
3.12 | 8.86 | 103.71 | 3.2 | |
6.25 | 12.01 | 103.68 | 3.4 |
Table 1.
Detection of AA in real juice sample
Catalyst | Substrate | Ref. | |
---|---|---|---|
BiW9Cu3 | TMB | 0.29 | [ |
CeO2 | 0.8-3.8 | [ | |
Hg2+/Citrate-AgNPs | 0.23 | [ | |
N-CQDs | 0.515 | [ | |
Cu-Ag/rGO | 0.634 | [ | |
Cu NPs | 1.047 | [ | |
Lysozyme-PtNPs | 0.63 | [ | |
Fe3O4@C | 0.38 | [ | |
Pt-Au DNPs | 0.22 | This work |
Table 2.
Comparative table of steady-state kinetic parameter for Pt-Au DNPs and other materials
Catalyst | Linear range/(μmol·L-1) | LOD/(μmol·L-1) | Ref. |
---|---|---|---|
FeCo NPs@PNC | 0.5-28 | 0.38 | [ |
N-CQDs | 5-40 | 1.773 | [ |
Cu-Ag/rGO | 1-30 | 3.6 | [ |
Cu NPs | 1-10 | 0.68 | [ |
LaF3:Ce,Tb | 8-10 | 2.4 | [ |
CuO/Pt | 1-600 | 0.796 | [ |
MIL-88 | 2.57-10.1 | 1.03 | [ |
Pt-Au DNPs | 1-15 | 0.078 | This work |
Table 3.
Comparison of earlier reports for the AA detection.
[1]CHAI D H, MA Z, YAN H, et al. Synergistic effect of sandwich polyoxometalates and copper-imidazole complexes for enhancing the peroxidase-like activity. RSC Advances, 2015, 5: 78771-78779.
[1]ASATI A, SANTRA S, KAITTANIS C, et al. Oxidase-like activity of polymer-coated cerium oxide nanoparticles. Angewandte Chemie-International Edition, 2009, 48(13): 2308-2312.
[2]WANG G L, XU X F, CAO L H, et al. Mercury(II)-stimulated oxidase mimetic activity of silver nanoparticles as a sensitive and selective mercury(II) sensor. RSC Advances, 2014, 4(12): 5867-5872.
[3]YADAV P K, SINGH V K, CHANDRA S, et al. Green synthesis of fluorescent carbon quantum dots from azadirachta indica leaves and their peroxidase-mimetic activity for the detection of H2O2 and ascorbic acid in common fresh fruits. ACS Biomaterials Science & Engineering, 2019, 5(2): 623-632.
[4]DARABDHARA G, SHARMA B, DAS M A, et al. Cu-Ag bimetallic nanoparticles on reduced graphene oxide nanosheets as peroxidase mimic for glucose and ascorbic acid detection. Sensors and Actuators B-Chemical, 2017, 238: 842-851.
[5]WANG N, LI B, QIAO F, et al. Humic acid-assisted synthesis of stable copper nanoparticles as a peroxidase mimetic and their application in glucose detection. Journal of Materials Chemistry B, 2015, 3: 7718-7723.
[6]Yu C J, CHEN T H, JIANG J Y, et al. Lysozyme-directed synthesis of platinum nanoclusters as a mimic oxidase. Nanoscale, 2014, 6(16): 9618-9624.
[7]AN Q, SUN C, LI D, et al. Peroxidase-like activity of Fe3O4@carbon nanoparticles enhances ascorbic acid-induced oxidative stress and selective damage to PC-3 prostate cancer cells. ACS Applied Materials & Interfaces, 2013, 5(24): 13248-13257.
[8]WU T, MA Z, LI P, et al. Colorimetric detection of ascorbic acid and alkaline phosphatase activity based on the novel oxidase mimetic of Fe-Co bimetallic alloy encapsulated porous carbon nanocages. Talanta, 2019, 202: 354-361.
[9]MI C, WANG T, ZENG P, et al. Determination of ascorbic acid via luminescence quenching of LaF3:Ce,Tb nanoparticles synthesized through a microwave-assisted solvothermal method. Analytical Methods, 2013, 5(6): 1463-1468.
[10]WANG X, HAN Q, CAI S, et al. Excellent peroxidase mimicking property of CuO/Pt nanocomposites and their application as an ascorbic acid sensor. Analyst, 2017, 142: 2500-2506.
[11]GAO C, ZHU H, CHEN J, et al. Facile synthesis of enzyme functional metal-organic framework for colorimetric detecting H2O2 and ascorbic acid. Chinese Chemical Letters, 2017, 28: 1006-1012.
References
[2] J LIU, X HU, S HOU et al. Au@Pt core/shell nanorods with peroxidase- and ascorbate oxidase-like activities for improved detection of glucose. Sensors and Actuators B-Chemical, 166, 708-714(2012).
[11] K VYADA P, K SINGH V, S CHANDRA et al. Green synthesis of fluorescent carbon quantum dots from azadirachta indica leaves and their peroxidase-mimetic activity for the detection of H2O2 and ascorbic acid in common fresh fruits. ACS Biomaterials Science & Engineering, 5, 623-632(2019).
[15] S ARAYSCHUKIAT, C KONGTES, A BARTHEL et al. Ascorbic acid as a bifunctional hydrogen bond donor for the synthesis of cyclic carbonates from CO2 under ambient conditions. ACS Sustainable Chemistry & Engineering, 5, 6392-6397(2017).
[30] M TSUJI, P JIANG, S HIKINO et al. Toward to branched platinum nanoparticles by polyol reduction: a role of poly(vinylpyrrolidone) molecules. Colloids and Surfaces a-Physicochemical and Engineering Aspects, 317, 23-31(2008).
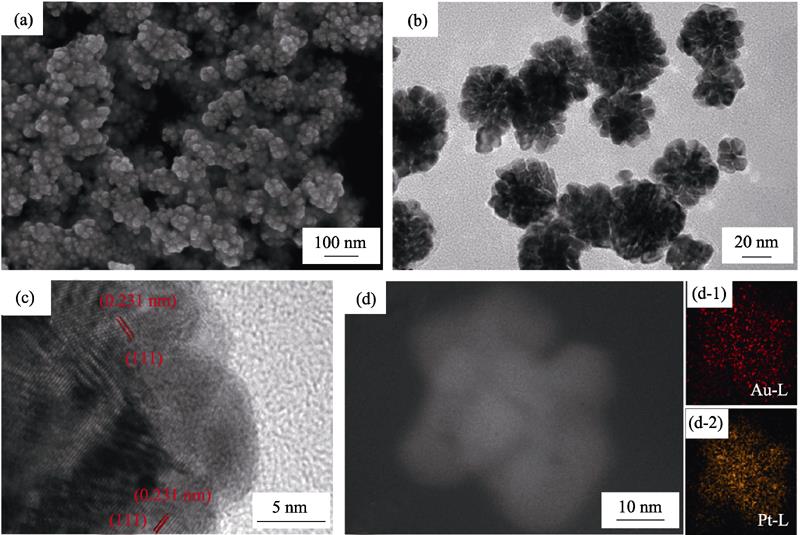
Set citation alerts for the article
Please enter your email address