Zijian Liu, Yuying Xi, Wenbo Zeng, Ting Ji, Wenyan Wang, Sitong Guo, Linlin Shi, Rong Wen, Yanxia Cui, Guohui Li, "Lead-free perovskite Cs2AgBiBr6 photodetector detecting NIR light driven by titanium nitride plasmonic hot holes," Photonics Res. 12, 522 (2024)

Search by keywords or author
- Photonics Research
- Vol. 12, Issue 3, 522 (2024)
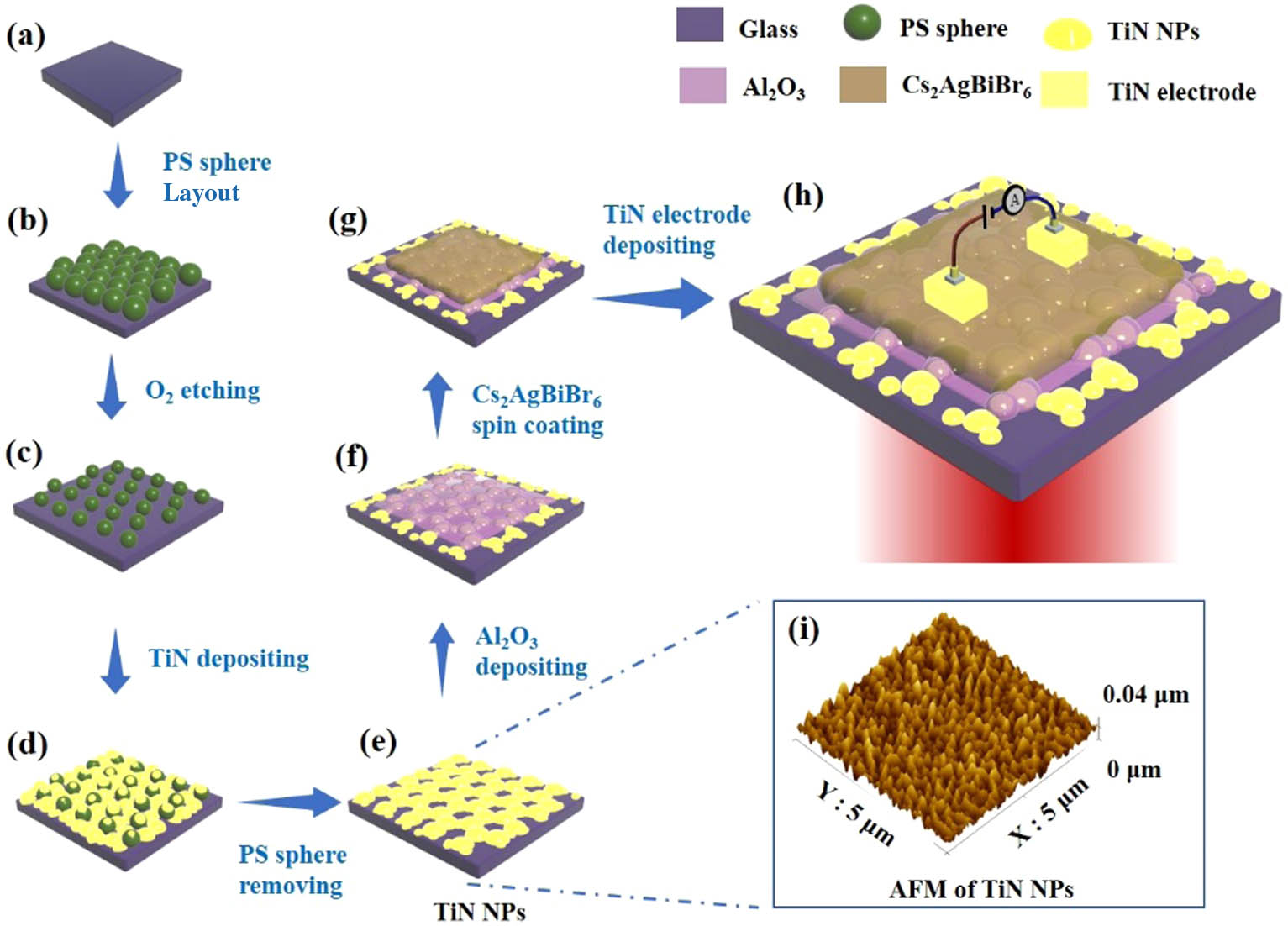
Fig. 1. Schematic flow chart illustrating the fabrication process of the plasmonic perovskite Cs 2 AgBiBr 6 PD incorporated with TiN NPs and Al 2 O 3 ultrathin layer. (a) Preparing a hydrophilic glass substrate; (b) forming a single layer of highly ordered closely packed PS nanospheres on glass substrate obtained by self-assembly method; (c) obtaining sparsely distributed PS nanosphere array by the RIE method; (d) depositing a TiN layer with a thickness of 40 nm by the RF magnetron sputtering method; (e) obtaining TiN NP array on the glass substrate after removing PS spheres; (f) depositing an ultrathin Al 2 O 3 layer on the above formed TiN NPs by ALD; (g) spin coating the Cs 2 AgBiBr 6 thin film on the Al 2 O 3 interfacial layer; and (h) sputtering TiN electrodes with a thickness of 80 nm on Cs 2 AgBiBr 6 using a copper mesh as the mask. In (h), the light is incident on the PD from the glass side. (i) 3D AFM image of the obtained TiN NPs.
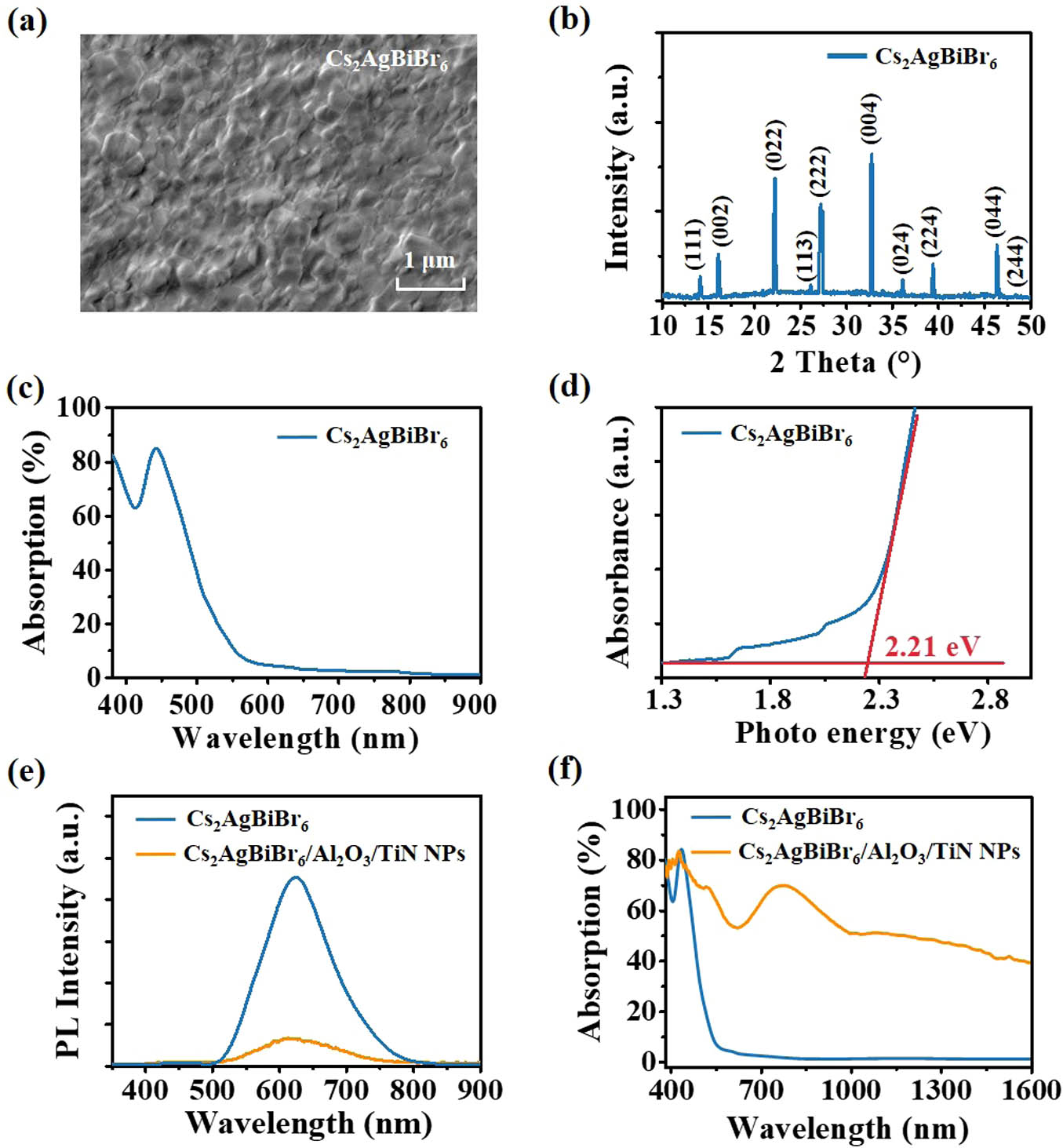
Fig. 2. (a) SEM image; (b) XRD pattern and (c) absorption spectrum of the Cs 2 AgBiBr 6 film; (d) absorption versus photo energy of the Cs 2 AgBiBr 6 film for deriving the bandgap; (e) steady-state PL spectra and (f) measured absorption spectra of the prepared films of Cs 2 AgBiBr 6 and Cs 2 AgBiBr 6 / Al 2 O 3 / TiN NPs, respectively.
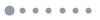
Fig. 3. (a) I –V curves of Cs 2 AgBiBr 6 , Cs 2 AgBiBr 6 / TiN NPs, and Cs 2 AgBiBr 6 / Al 2 O 3 / TiN NP devices measured in the dark. (b) and (c) I –V curves of the Cs 2 AgBiBr 6 , Cs 2 AgBiBr 6 / TiN NP, and Cs 2 AgBiBr 6 / Al 2 O 3 / TiN NP devices under 505 and 850 nm light illuminations. Insets, transient photocurrent responses of the Cs 2 AgBiBr 6 and Cs 2 AgBiBr 6 / Al 2 O 3 / TiN NP devices at 505 and 850 nm, respectively; (d) transient photocurrent curves of the Cs 2 AgBiBr 6 device and Cs 2 AgBiBr 6 / Al 2 O 3 / TiN NP device at 505 and 850 nm; (e) LDR characterization of the Cs 2 AgBiBr 6 / Al 2 O 3 / TiN NP device measured under 505 and 850 nm illuminations at 1 V; (f)–(h) EQE, R , and D * of the Cs 2 AgBiBr 6 and Cs 2 AgBiBr 6 / Al 2 O 3 / TiN NP devices at different wavelengths; (i) measured noise currents of the Cs 2 AgBiBr 6 and Cs 2 AgBiBr 6 / Al 2 O 3 / TiN NP devices at various frequencies at 1 V bias. The calculated shot noise and thermal noise limits are also included for reference.
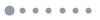
Fig. 4. (a) EQE and absorption enhancement factors at different wavelengths; (b) simulated absorption spectra of the Cs 2 AgBiBr 6 and Cs 2 AgBiBr 6 / TiN NP structures; (c) electric field distributions in the x − y plane at the simulated absorption peak of 735 nm for the Cs 2 AgBiBr 6 / TiN NP structure; (d) contact potential map of the Al 2 O 3 modified TiN NPs taken by the KPFM technique (φ and W are the average contact potential and work function of the Al 2 O 3 modified TiN NPs, respectively); (e) energy band diagram of the Cs 2 AgBiBr 6 / Al 2 O 3 / TiN NP device (W TiN NPs = 4.92 eV , E c = − 3.6 eV , E v = − 5.7 eV , ϕ b = 0.78 eV ); (f) working mechanism of the Cs 2 AgBiBr 6 / Al 2 O 3 / TiN NP device, illustrating hot hole excitation and transfer driven by plasmonic resonances.
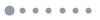
Fig. 5. Atomic force microscopy (AFM) image of the (a) monolayer polystyrene (PS) spheres and (b) sparsely-distributed PS nanosphere array.
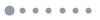
Fig. 6. SEM images of Cs 2 AgBiBr 6 films prepared at the acceleration speed of (a) 1400 (r/min)/s, (b) 1200 (r/min)/s, (c) 1000 (r/min)/s, and (d) 800 (r/min)/s, respectively.
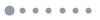
Fig. 7. (a) 3D AFM and (b) KPFM images of the Cs 2 AgBiBr 6 film (φ and W are the average contact potential and work function of the Cs 2 AgBiBr 6 , respectively).
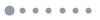
Fig. 8. XRD patterns of the Cs 2 AgBiBr 6 films prepared on the (a) surface of Al 2 O 3 modified TiN NPs and (b) directly prepared on top of TiN NPs.
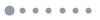
Fig. 9. Transient photocurrent characteristic of the devices switched on and off multiple times at a power density of 10.20 mW / cm 2 : (a) the Cs 2 AgBiBr 6 device at 505 nm, (b) the Cs 2 AgBiBr 6 / Al 2 O 3 / TiN NPs device at 505 nm, (c) the Cs 2 AgBiBr 6 / Al 2 O 3 / TiN NPs device at 850 nm.
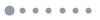
Fig. 10. LDR characterization of the Cs 2 AgBiBr 6 device under 505 nm LED measured at 1 V.
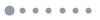
Fig. 11. I − V curves of (a) Cs 2 AgBiBr 6 and (b) Cs 2 AgBiBr 6 / Al 2 O 3 / TiN NPs devices measured in dark and under illumination (λ = 1310 nm and 1550 nm).
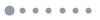
Fig. 12. (a) Particle size distributions of the TiN NPs. (b) Structure of the Cs 2 AgBiBr 6 / TiN NPs device utilized in simulation. (c) Measured and simulated absorption spectra of the Cs 2 AgBiBr 6 / Al 2 O 3 / TiN NPs structure. (d) Electric field distributions at multiple x − z planes at the simulated absorption peak of 735 nm. (e), (f) Electric field distributions in the x − y plane at the simulated absorption peaks of (e) 555 nm and (f) 1145 nm.
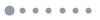
Fig. 13. Contact potential map of the reference highly oriented pyrolytic graphite (HOPG) sample taken by the KPFM technique (φ and W are the average contact potential and work function of the HOPG, respectively).
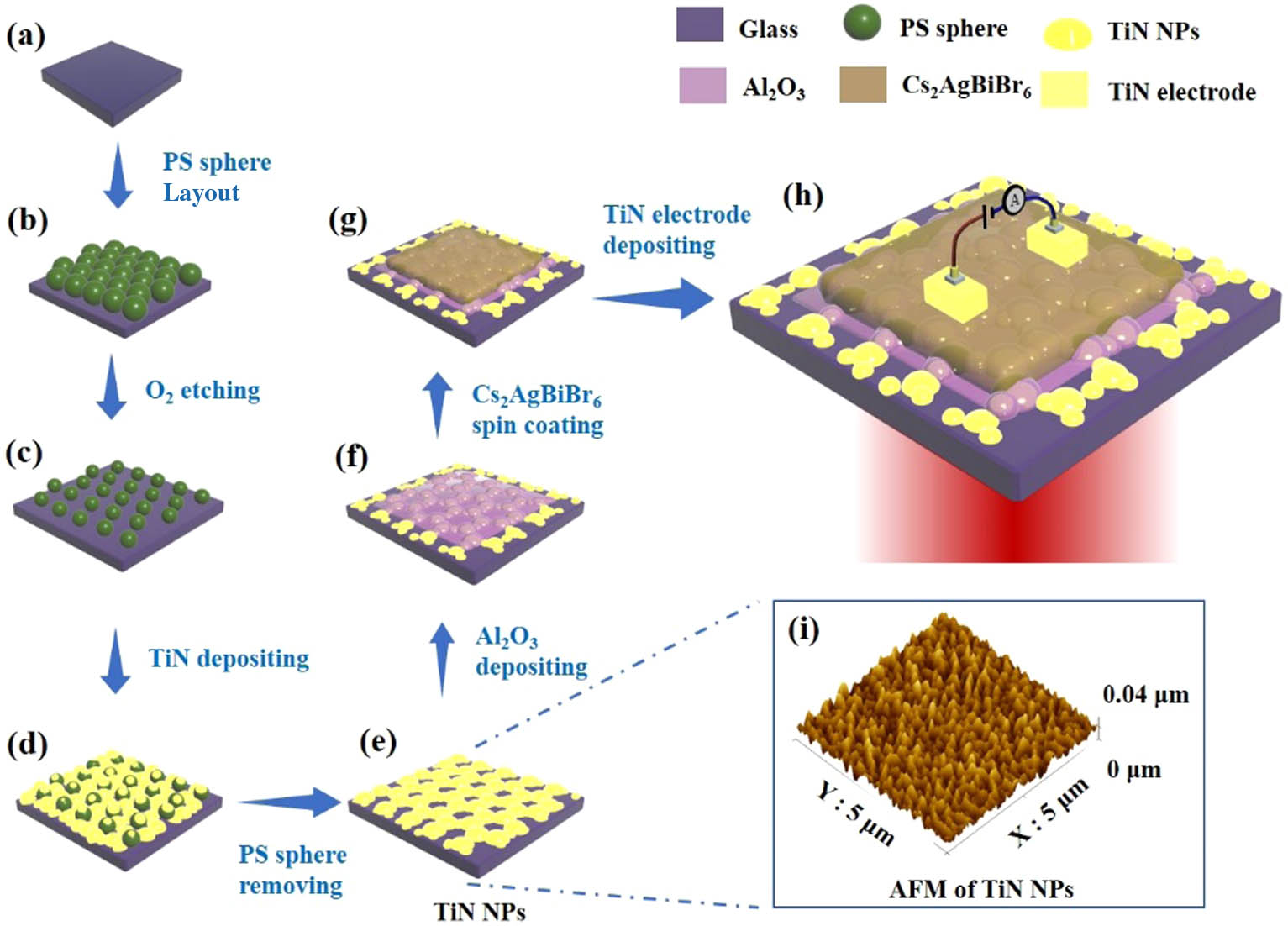
Set citation alerts for the article
Please enter your email address