Yu He, Xingfeng Li, Yong Zhang, Shaohua An, Hongwei Wang, Zhen Wang, Haoshuo Chen, Yetian Huang, Hanzi Huang, Nicolas K. Fontaine, Roland Ryf, Yuhan Du, Lu Sun, Xingchen Ji, Xuhan Guo, Yingxiong Song, Qianwu Zhang, Yikai Su, "On-chip metamaterial-enabled high-order mode-division multiplexing," Adv. Photon. 5, 056008 (2023)

Search by keywords or author
- Advanced Photonics
- Vol. 5, Issue 5, 056008 (2023)
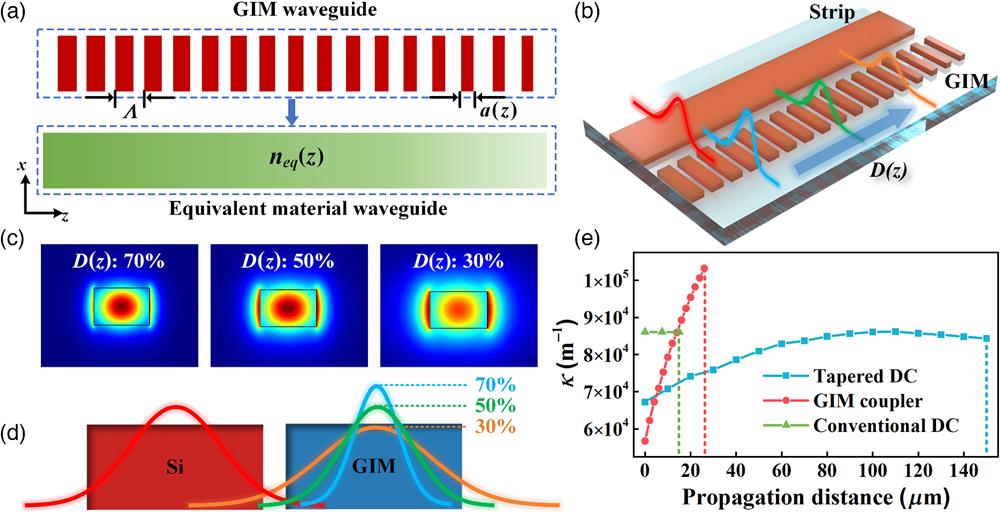
Fig. 1. Planar-metamaterial-enabled refractive-index-distribution manipulation and GIM-enabled adiabatic coupling. (a) Conceptional illustration of a 1D metamaterial structure with a linearly decreasing duty cycle , which can be regarded as a strip waveguide with a varying refractive index . (b) Schematic configuration of a GIM-based mode coupling process. The GIM waveguide is tailored with a linear duty cycle distribution along the propagation direction. (c) Simulated electric field distributions of GIM waveguides with different duty cycles. (d) Cross-section view of the GIM-based direction coupler. The Gaussian curves illustrate the power distributions of the modes supported by the GIM waveguide. (e) Calculated coupling coefficients for the tapered DC, GIM coupler, and conventional DC as functions of the propagation distance.
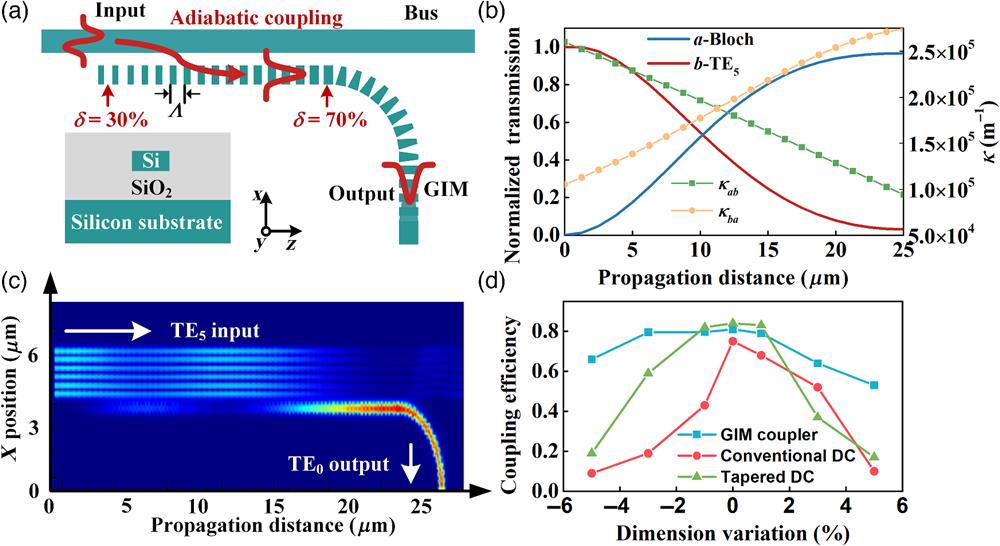
Fig. 2. GIM-based coupler for 16-channel MDM. (a) Schematic configuration of the GIM-based coupler designed for selective mode coupling. The duty cycle of the GIM waveguide linearly increases from 30% to 70% along the propagation direction for constructing a linear refractive index distribution. (b) Calculated coupling coefficients and and the normalized transmission responses for the mode in the strip waveguide (mode ) and the Bloch mode in the GIM waveguide (mode ), as a function of propagation distance. (c) Simulated electric field distribution for the mode demultiplexing. (d) Simulated mode demultiplexing process using GIM structure, conventional DC, and tapered DC, respectively, when the waveguide dimension varies.
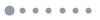
Fig. 3. GIM-based couplers for 16-channel MDM. (a) Schematic configuration of the 16-channel mode MUX. (b) Microscope image and (c) SEM image of the 16-channel GIM-based mode MUX and deMUX. (d)–(f) Magnified SEM images of the coupling regions.
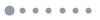
Fig. 4. (a)–(p) Measured transmission responses of the 16-channel mode deMUX. Each subplot shows the transmission and crosstalk recorded at 16 output ports for a selected input channel.
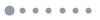
Fig. 5. High-speed data transmission experiment. (a) Experimental setup for the high-speed transmission experiment of the 16-channel MDM chip. The insets show the photographs of the testing platform and the fiber array-based vertical coupling. (b) Monitored optical spectrum of the recombined signal at the receiver side. (c) Calculated BERs of 40-GBaud 16-QAM signals for 16 modes after MIMO-FFE and MLSD. All channels are below the 15% FEC threshold. (d) Recovered constellations for all the 16 modes.
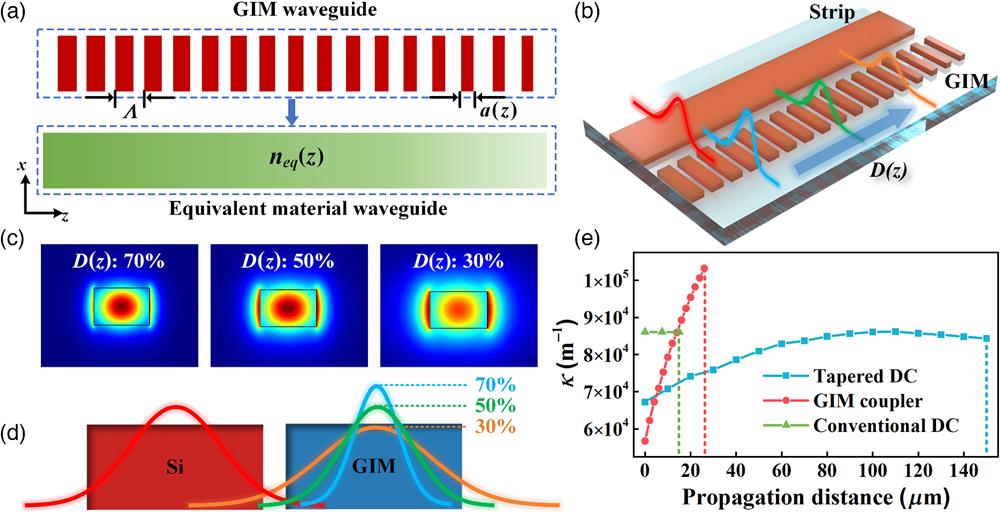
Set citation alerts for the article
Please enter your email address