Author Affiliations
1Pohang University of Science and Technology, Department of Mechanical Engineering, Pohang, Republic of Korea2Pohang University of Science and Technology, Department of Chemical Engineering, Pohang, Republic of Korea3POSCO–POSTECH–RIST Center for Flat Optics and Metaphotonics, Pohang, Republic of Koreashow less
Fig. 1. Tunable metalenses by light source. (a) Schematic illustration of spin-decoupled metalenses.
69 (b) Simulated and experimental electric field intensity distributions at line
for multiple polarization states of incident light (LCP, LECP, LP, RECP, RCP from left to right).
69 (c) Schematic of spin selective metalenses that can focus RCP and LCP incident beams to different focal points.
70 (d) Schematic view of metalenses using the combination of the PB phase and propagation phase.
71 (e) Schematic of step-zoom metalenses in which the focal length is changed according to the linear polarization of the incident light.
72 (f) Schematic of metalenses doublet that has different functions depending on the polarization of the incident light.
73 Fig. 2. Tunable metalenses by electrical bias. (a) Schematic view of LC integrated metalenses that change functionality achromatic focusing to dispersive focusing when applying voltage bias to LCs.
78 (b) Side view of TN LCs integrated electrically tunable metalenses. It modulates the polarization state of the incident beam depending on the applied voltage bias.
79 (c) Schematic of a focus tunable graphene metalens when DC voltage bias is applied to this metalens.
85 (d) Variation of focal length and focal spot intensity when the design is a packed pattern (top) and shifted bezel pattern (bottom).
85 (e) Schematic of tunable graphene metalenses in which the chemical potential of graphene is controlled by applying a gate voltage.
88 (f) Schematic view of electrically tunable metalenses in which the refractive index of BTO antennas is changed by applying voltage bias.
90 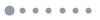
Fig. 3. Tunable metalenses by non-electrical input. (a) Schematic of metalenses that are tuned mechanically by stretching the substrate to tune the focal length.
96 (b) Measured longitudinal beam profiles according to different stretch ratios (top), intensity distributions of transmitted cross polarized light with different stretch ratios (bottom left), measured and calculated stretch ratio-focal length graph (bottom right).
96 (c) Schematic illustration of metalenses that are tuned mechanically by rotating the substrate (left) and phase distribution of one of two lenses (right).
97 (d) Schematic view of a tunable metalenses system that consists of two cubic metasurfaces.
98 (e) Schematic illustration of MEMS tunable metalenses in which the focal length is tuned by controlling the distance between two lenses.
99 (f) Schematic illustration of varifocal metalenses in which the focal length is tuned by exploiting the phase change of GSST by furnace annealing.
100 (g) Schematic of varifocal metalenses using phase-change material
. The phase of
is changed by controlling the temperature, and the change affects the focal length.
101 Fig. 4. Tunable metaholograms by light source. (a) Schematic of tunable metaholograms that generate dual images that can be changed by the polarization state of an incident light beam. (b) Experimentally obtained holograms at incident wavelengths of 24 nm (left) and 475 nm (right).
129 (c) Design principle of OAM multiplexing metaholograms and metahologram images with a planar wavefront (
). (d) Reconstructed images using four different OAM beams with topological charges
,
, 1, and 2.
137 (e) Illustration of a video metahologram using OAM. (f) It changes their images depending on the angular momentum of the incident light.
54 (g) Schematic of the reprogrammable metaholograms with the modulated incident beam by SLM. (h) The recorded images with the characterized incident light (left), with uniform laser light (middle), and the incident laser beam itself (right).
140 Fig. 5. Tunable metaholograms by electrical bias. (a) Schematic of three major states of LC (nematic, smectic, isotropic). (b) Design and demonstration of electrically tunable dielectric metasurfaces that use LCs.
149 (c) Working principle of the electrically controlled digital metasurface device (DMSD). (d) SEM image of the DMSD and experimental results by independent control of seven electrodes.
150 (e) Schematic of the bifunctional vectorial metasurfaces with LC analyzer. (f) SEM image of fabricated metasurfaces, and metaholograms that can be tuned by applying different voltages [scale bars: (left)
, (right)
].
152 Fig. 6. Tunable metaholograms by non-electrical input. (a) Changing phase geometry (amorphous, semicrystalline, crystalline) that can construct SAM-OAM conversion by tuning the crystallization level of GST. (b) SEM images of fabricated metasurfaces and two different metaholographic images in response to three crystallization levels (top: RCP, bottom: LCP).
158 (c) Schematic of the hydrogenating metasurfaces. (d) Four different holographic images during two hydrogenation and two dehydrogenation processes.
160 (e) Illustration of the surface pressure-responsive designer LC; (f) experimental results before (left, LCP) and after (right, RCP) surface pressure by touch of finger.
164 (g) Schematic of a gas sensor with LC-integrated metahologram. (h) The gas sensor changes optical images when hazardous gas is detected.
34 Types | Metalenses | Metaholograms | Research goals | Multi-, varifocal length69–89,91–118 | Storing multiple images54,129–142 | Visualized sensing (thermal, chemical, etc.)155–162 | Optical encryption140–142 | Common stimuli | Electrically tunable LC76–82,149–155 | Polarization (circular and linear polarization)69–75,129–135 | Mechanical (strain, relative position of two metasurfaces)96–99,102–114,163,164 | Vectorial metasurfaces132,133 | Different stimuli | Electrically tunable 2D materials83–89,91–95 (graphene, etc.) | Topological charges of OAM136–139 | Chemically/Thermally tunable holograms155–162 | Pressure responsive LC34,164 | Common problems/challenges of controlling light source | Tradeoff relation between efficiency and design simplicity | Reacting with subtle polarization state differences | RGB OAM hologram and ultrafast switching | Common problems/challenges of electrical bias | Hard to control meta-atoms locally | Local control of nano pixels and integrating them with metasurfaces | Tradeoff between the number of images and their quality (efficiency) | Common problems/challenges of non-electrical input | Need to mechanically move parts in case of stretching methods | Long phase transition time and optimizing design process | Long phase transition time and limited operating bandwidth in infrared regime in case of using PCMs |
|
Table 1. Summary of tunable metalenses and metaholograms.