Abstract
The rapid spread of viral zoonoses can cause severe consequences, including huge economic loss, public health problems or even global crisis of society. Clinical detection technology plays a very important role in the prevention and control of such zoonoses. The rapid and accurate detection of the pathogens of the diseases can directly lead to the early report and early successful control of the diseases. With the advantages of being easy to use, fast, portable, multiplexing and cost-effective, semiconductor biosensors are kinds of detection devices that play an important role in preventing epidemics, and thus have become one of the research hotspots. Here, we summarized the advances of semiconductor biosensors in viral zoonoses detection. By discussing the major principles and applications of each method for different pathogens, this review proposed the directions of designing semiconductor biosensors for clinical application and put forward perspectives in diagnostic of viral zoonoses.The rapid spread of viral zoonoses can cause severe consequences, including huge economic loss, public health problems or even global crisis of society. Clinical detection technology plays a very important role in the prevention and control of such zoonoses. The rapid and accurate detection of the pathogens of the diseases can directly lead to the early report and early successful control of the diseases. With the advantages of being easy to use, fast, portable, multiplexing and cost-effective, semiconductor biosensors are kinds of detection devices that play an important role in preventing epidemics, and thus have become one of the research hotspots. Here, we summarized the advances of semiconductor biosensors in viral zoonoses detection. By discussing the major principles and applications of each method for different pathogens, this review proposed the directions of designing semiconductor biosensors for clinical application and put forward perspectives in diagnostic of viral zoonoses.1. Introduction
Zoonoses are kinds of infectious diseases caused by the pathogens which are transmitted to humans from animals. These pathogens include viruses, bacteria, parasites and fungi. Among these pathogenic microorganisms, viral pathogens such as coronavirus, influenza virus, hepatitis B virus, Zika virus, human immunodeficiency virus (HIV), etc. have caused severe health problems and even death[1-5], making them more and more concerned (Fig. 1). Fast spreading and fatalities of these viruses cause serious impact to modern society. According to theChina Health Statistical Yearbook 2019 published by National Health Commission of the PRC[6], there were 1709 pathogens that cause human illness, of which 832 (49%) were zoonotic pathogens.
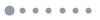
Figure 1.(Color online) Schematic process of the detection of biosensor. Samples are collected from animals, human, and environment, then the virus particles, antibodies, RNA or DNA are targeted and detected by semiconductor biosensors. The signals are finally displayed by visible image.
To prevent the spread of epidemic diseases, biological detection is one of the most important technologies for control measures especially in the early stage of the epidemic[1,3,5,7]. Traditional detection methods such as virus isolation, serological methods and molecular biological techniques show many disadvantages[7-11]. For example, virus isolation is time consuming, and required a high level of conditions from supporting laboratory, which is unable to perform on-site detection. The transport of the samples faces with the risk of further diffusion of potential positive samples. The failure to detect the disease on site may cause spread of the epidemic and unpredictable economic losses. In addition, the sensitivity and specificity of traditional methods still need to be further improved[12-14]. These problems may further extend the diagnosis time of positive cases, and increase the difficulty of epidemic prevention and control.
Biosensors can be defined as miniaturized devices incorporating recognition elements for identifying and/or quantifying either a synthetic or biochemical analyte. Semiconductor is a kind of material with conductivity between conductors and insulators at room temperature, which is widely used in fabricating diodes. For example, using semiconductors that are very sensitive to changes in external electric fields, researchers have constructed semiconductor field-effect transistors which could respond to different biological signals with high sensitivity and fast response. Besides, semiconductor biosensors have been demonstrated to have enormous recognizable fixation extends, high affectability, high consistency for solid detecting, and the ability to coordinate with other microfluidic gatherings. With these advantages, it can combine biotechnology with electronic technology or optical biology to develop detection methods, which began to emerge in the detection field as a newly developed subject[15-17]. Semiconductor biosensors have become one of the best choices for epidemic diseases testing and prevention with no doubt.
The typical semiconductor biosensors are majorly composed of the following parts: signal recognition element, signal conversion element and auxiliary element[18]. Among them, signal recognition element (especially the probes) and signal conversion element are the core elements of semiconductor biosensors (Fig. 2). A semiconductor has a few qualities such as warm affectability, photosensitivity, negative resistivity temperature, rectifiable, etc. Semiconductor materials can be utilized for signal conversion elements like control gadgets, optoelectronic gadgets, pressure sensors, thermoelectric refrigeration, and different applications other than assembling enormous scale coordinated circuits.
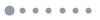
Figure 2.(Color online) Schematic representation of the major principles of semiconductor biosensors. (a) Schematic representation of the identification process, the key point is the recognition and conversion element. (b) Schematic representation of the major principles for optical biosensors. (c) Schematic representation of the major principles for electrochemical biosensors.
For the detection of viral zoonoses, the detection targets of semiconductor biosensors include nucleic acid, protein, virus particles and sometimes specific cytokines (Fig. 1). The design of the probes is very important, which closely influences the sensitivity and specificity of semiconductor biosensors. The process in which the probes interact specifically with the sample to generate biological signals (in the form of light, heat, pH change, etc.) is called biometric recognition. Then the biological signals are captured by signal recognition element and produce light or electric potential that further transmit the signal to conversion element[18]. The auxiliary elements then accept the signals and output the signals with a display screen (Fig. 1).
In this paper, the advances of semiconductor biosensor applied in detection of viral zoonoses were reviewed. Among the various nanomaterials used in semiconductor biosensors,graphene, carbon nanotube and silicon nanowire were most important and would be discussed in this article. Through the summary of the advance of the semiconductor biosensors applied in detection of viral zoonoses, the future development and prospects of semiconductor biosensors were proposed.
2. Semiconductor biosensors for detection of zoonoses
The main problem to be solved during the development of semiconductor biosensors is how to convert the weak biological signals into optical or electrochemical signals and amplify them (Fig. 2). For photoelectric semiconductor biosensors (Fig. 2(b)), the target molecule generates light energy during recognition and the light energy will be converted into electrical energy by photoelectric materials. Two electron flow directions are inside the semiconductor, namely electron–hole recombination and electron transfer. When using an n-type semiconductor as photoelectric material, the electrons in the conduction band will migrate to the electrode, and the electron donors in the electrolyte solution will provide a continuous supply of valence band. When using a p-type semiconductor as photoelectric material, the conduction band of electrons will be transferred to the electrolyte solution (Fig. 2(b)).
For electrochemical semiconductor biosensors, chemical reactions (like redox reactions) caused by the interaction of the bio-molecules would generate changes. In a threshold/source–drain voltage, it further causes the conductivity change of semiconductor, allowing for a higher sensitivity of the signals to be recorded and displayed (Fig. 2,Fig. 2(c)).
Table Infomation Is Not Enable
The semiconductor biosensors aimed at the detection of nucleic acid molecules of the pathogens are based on the principles of specific complementary pairing of nucleic acid, specific interaction between protein-nucleic acid, and specific interaction between chemical molecular-nucleic acid[19]. Besides nucleic acid, detection of the virus particles, antigens or special antibodies of virus are also necessary for the requirements of epidemiological investigation. Unlike diagnosis of human diseases, the proportion of pathogens is very low in the clinic samples. It is worth mentioning that the signal amplification methods are also frequently used by the researchers as a pretreatment method before being applied to the recognition element (Table 1). Auxiliary elements which can amplify the target molecules are often designed. Amplification methods included target recycling reaction, DNAzyme-based reaction, magnetosome amplification method[20]. Although the amplification of nucleic acid can effectively improve the sensitivity of the detection, the disadvantages of this operation are also very obvious, such as time-consuming, non-specific amplification of nucleic acid, also sometimes requirement of more experimental conditions.
Like semiconductor biosensors for detecting nucleic acids, semiconductor biosensors for detecting proteins are also composed of similar elements. The recognition element of detecting proteins is mainly based on the principle of protein interaction. According to different detection targets, the interaction can be divided into antigen-antibody, antibody-polypeptide, antigen ligand, etc. To a large extent, the sensitivity and specificity of the sensor are determined by the interaction force between the molecules. In addition, due to the instability of the protein, we must also consider whether the anchoring conditions affect the activity of the anchored protein.
2.1. Development of the capture probes
Normally, the biological signal generated in the interaction of nucleic acids or proteins is very low, and difficult to detect. As mentioned above, the principles of nucleic acid sequence pairing, not only DNA-DNA pairs, the DNA to DNA, RNA to DNA, DNA to peptide nucleic acid (PNA)[25–27], and RNA to PNA, etc., are the basic fundamental for design of the capture probes. Genetic sequences of primers and complementary capture probes are designed based on analysis of target virus genome. Then, immobilized DNA probes as a capture element, allowing specific hybridization reactions to target DNA or target RNA molecule. In order to improve the specificity and sensitivity of semiconductor biosensors, probe molecules are often modified, such as fluorescence modification, chemical molecular modification, or nano-material modification, so that more obvious signals can be released during the detection process[28,29].
For consideration of high selectivity, stability and cost-effectiveness, new recognition elements, such as nucleic acid aptamers or peptides were considered. Nucleic acid aptamer is a kind of oligonucleotide sequence synthesized and screened in vitro, which is rolled and folded to form a specific tertiary structure with certain functions, and can form complexes with corresponding nucleic acids through complexation.
2.2. Advances of nanomaterials applied in semiconductor biosensors
For the advantages of large specific surface area, strong adsorption capacity and good directional ability, nanoparticles have become a research and development hot-spot in recent years. Common nanomaterials include carbon nanomaterial (carbon nanotubes, grapheme and carbon black)[30], silicon nanomaterial and metallic oxide nanomaterial, etc. These nanomaterials could be also classified as formally zero- (0D), one- (1D) and two- (2D) dimensional nanomaterials. The semiconductor biosensors based on graphene, carbon nanotube, and silicon nanowire are further discussed below.
2.2.1. Advance in graphene-based biosensors
Graphene is an atomically thin layer of sp2 carbon atoms covalently connected into a honeycomb lattice with a unique electronic structure, making it a semimetal/zero-gap semiconductor. Graphene shows the characteristics of extremely electronically and thermally conductive, chemically stable, strong, flexible, highly optically transparent but impenetrable to any molecule. It has various forms such as graphene oxide (GO), reduced graphene oxide (rGO), graphene nanoribbons (GNRs) and so on, which have received worldwide attention for the development of biosensors.
Four principles are utilized when graphene is used to sense biomolecules in biosensors: electron transfer materials, electrochemical impedance materials, as field effect transistors (FETs) and as photon/phonon transfer materials. The essence during the detection of the signals is the change of the conductivity of graphene which is caused by charge under different electric field or light excitation conditions. For example, in back gate FETs, the change in a threshold/source-drain voltage causes the conductivity of graphene to change, allowing for a higher sensitivity to be recorded (Fig. 3). In liquid gates FETs, graphene is very susceptible to a change in the surrounding liquid environment, whether it is by ion density or a surface charge, which produces a higher detection sensitivity compared to other non-graphene liquid gate FET biosensors. Based on this principle, different sensing mechanisms including optical, electrochemical or electrical can be employed with graphene-based biosensors (Fig. 3).
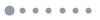
Figure 3.(Color online) Schematic representation of the major principles of graphene based semiconductor biosensors. (a) Representation of the major principles of graphene FET biosensors. (b) Representation of the major principles of graphene based optical biosensors.
For the electrochemical biosensors based on graphene, signals are collected and transmitted under the action of an external electric field. For the optical biosensors based on graphene, the performance of the device relies upon the interaction between a recognition element and the optical transducer. Optical biosensors can be characterized into two general categories: label-based and label-free sensors.
For label-based sensors, they require the employment of labels, such as colorimetric, fluorescent, or luminescent, to produce the light signals and the target signals are identified using fluorescence microscopy or fluorescence spectrometry. For the label-free sensors, the detected signal is the result of the direct interaction of the sample with the immobilized recognition element on the transducer’s surface. In the literatures published in recent 5 years, graphene-based biosensors have played a vital role in the detection of different types of viruses, such as Ebola virus, influenza virus, AIDS (HIV), hepatitis B virus (HBV), and hepatitis C virus (HCV), etc (Table 2).
Table Infomation Is Not Enable
The influenza virus consists of a single-stranded RNA genome and several surface antigens—for instance, hemagglutinin (H) and neuraminidase (N). As reported, based on rGO materials, the limit of detection (LOD) of the detection of influenza virus was 33 PFU/mL for electro biosensors, and the LOD of the detection was 3.8 pg/mL for optical biosensor[42] (Table 2).
Ebola virus disease, widely known as a fatal disease, can cause severe infection. Two research groups, Jinet al. and Maityet al.[43,44] developed rGO based electro biosensors, the LOD of the biosensors were 2.4 pg/mL and 1μg/mL respectively (Table 2).
For HIV/AIDS which cause a large number of patients over the world, ultrasensitive diagnostic techniques for the early detection of HIV during the infection cycle are required. The LOD base on G/CVD was 0.1 ng/mL, and the LOD base GO/PANi was 100 aM[53,54] (Table 2).
The hepatitis C virus (HCV) is the most widespread cause of chronic liver disease, affecting 2%–3% of the world population. In the past, early detection of HCV was difficult, especially for those living in underdeveloped nations. Jialonget al. employed reduced graphene oxide nanosheets (rGONS) with the hybridization chain reaction (HCR) amplification technique, to develop an ultrasensitive method for the detection of HCV RNA. The reported LOD was as low as 10 fM, which is substantially lower than the commonly used fluorescence approach based on GO (Table 2).
HBV is a hepadnaviridae virus that has its viral double-stranded DNA enclosed. Hepatitis B virus core antigen (HBcAg) is the major structural protein. Abd Muain MFet al.[55] have demonstrated that the immobilization of HBcAg onto the gold nanoparticles-decorated reduced graphene oxide (rGO-en-AuNPs) nanocomposite could be used as an antigen-functionalized surface to sense the presence of anti-HBcAg (Table 2). The electrochemical response showed a linear relationship between the resistance of electron transfer and the concentration of anti-HBcAg, ranging from 3.91 to 125.00 ng/mL and with the limit of detection (LOD) of 3.80 ng/mL at 3 σ/m[55].
2.2.2. Advance in carbon nanotube-based semiconductor biosensors
Carbon nanotubes (CNTs) and graphene are graphite materials, but different in the arrangement and combination of carbon atoms. CNTs are seamless one-dimensional tubular structures formed by the curling of two-dimensional graphene. Each carbon atom on the tube wall forms a hexagonal coaxial carbon ring structure with surrounding carbon atoms through sp2 hybridization and a small amount of sp3 hybridization.
According to the number of graphene layers curled to form carbon nanotubes, carbon nanotubes can be divided into single-wall carbon nanotubes and multi-wall carbon nanotubes. Their diameters are generally 0.6–20 nm, and the spacing between the layers constituting carbon nanotubes is about 0.34 nm[31]. Carbon nanotubes have high modulus, high strength and unique electrical properties, light weight, high thermal conductivity, unique electrocatalytic action, minimal surface fouling and high surface to volume ratio,which make them excellent semiconductor materials.
With the similar principles to the graphene, CNTs have been applied to a wide variety of different types of biosensors which can be categorized according to the type of transduction mechanism, the most common classifications being optical and electrochemical (majorly field-effect-based) sensors.
For optical sensors, optical transduction mechanisms are commonly used for biosensors as they are capable of high sensitivity, stability and are suitable for multiplex detection. CNTs have fluorescent properties that are highly dependent on their physical structure, and consequently have been widely utilized in fluorescent biosensors (Table 3). Rebeccaet al.[34] introduced a single-walled carbon nanotube (SWCNT)-based optical sensing approach. They further demonstrated that these ACE2-SWCNT nanosensors retained sensing capacity in a surface-immobilized format, exhibiting a 73% fluorescence turn-on response within 5 s of exposure to 35 mg/L SARS-CoV-2 virus-like particles[34].
Table Infomation Is Not Enable
For electrochemical CNT biosensors, the CNTs can be deposited onto the electrodes via methods such as drop-casting or by direct synthesis of the CNTs onto the electrode surface (Table 3). Shaoetal.[37] used a high-purity semiconducting single-walled carbon nanotube (SWCNT)-based field-effect transistor (FET) decorated with specific binding chemistry to assess the presence of SARS-CoV-2 antigens in clinical nasopharyngeal samples[37]. The results showed that it could reach a limit of detection of 0.55 fg/mL for spike antigen and 0.016 fg/mL for N antigen in calibration samples.
2.2.3. Advance in silicon nanowire-based biosensors
The silicon nanowire (SiNW) is very sensitive to a small amount of surface charge changes, which would further cause significant changes of its conductivity. The biological probe molecule is fixed on the surface of SiNW by chemical modification, and the target molecule is specifically bound to the surface probe molecule. When the distance from the probe molecule to the SiNW surface is greater than the Debye length, the signal combining the probe molecule with the target is shielded, and the conductivity of SiNW channel does not change (Fig. 4). When the target molecule specifically combines with the surface probe molecule, then the distance from the probe molecule to the SiNW surface is less than the Debye length. In this circumstance, it can cause the conductivity change of the semiconductor channel through capacitive coupling (Fig. 4). In this process, biological signals are successfully converted into electrical signals that can be amplified and detected.
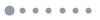
Figure 4.(Color online) Schematic representation of the major principle of silicon nanowire based semiconductor biosensor.
As a kind of important one-dimensional semiconductor nanomaterials, silicon nanowires have widely application prospects in high-performance field effect transistors, single electron detectors and field emission elements due to their unique properties such as fluorescence and ultraviolet, emission and electron transport, heat conduction, high surface activity and quantum confinement effects. In recent 5 years, several research has been focused on the development of nanowire based biosensors in detection of viral zoonoses, which refers to the hepatitis B virus, Influenza virus, and SARS-CoV-2, etc. (Table 4). Silicon nanowire field effect transistor and polycrystalline silicon nanowire field-effect transistors were the commonly used methods which were applied in detection of viral zoonoses.
Table Infomation Is Not Enable
Among the field effect transistor (FET) sensors, the silicon nanowire field effect transistor (SiNWFET) sensor has attracted significant attention. The well-developed silicon industry offers great benefits to the study of SiNWFET. Many studies have demonstrated the potential applications of SiNWFET, including detection of nucleic acids, antibodies, antigens, and proteins. These findings showed the benefits of SiNWFET, including ultrahigh-sensitivity, real-time, and label-free detection, making it a great potential candidate in biosensor development.
In the report, Anik has fabricated the polycrystalline silicon nanowire field-effect transistors (pSiNWFETs) through the sidewall spacer etching technique. Furthermore, the chemical functionalization techniques of the pSiNWFET surface were performed for the immobilization of the relevant antibodies for sensing the HBV-related biomarkers such as HBsAg and HBx proteins. These semiconductor biosensors can detect the HBsAg and HBx proteins at the femto-molar level providing an opportunity to evaluate and understand hepatitis infection[40,57]
Uhmeet al.[58] demonstrated that the ultrasensitive electrical detection of the HA1 domain of hemagglutinin (HA), a representative viral surface protein of the influenza virus, using the top-down complementary metal oxide semiconductor (CMOS) processed silicon nanowire (SiNW) field-effect transistor (FET) configuration. Femtomolar concentrations of HA1 can be detected (ΔVT = 63 mV/dec, ΔVT = 112 mV at 1 fM HA1) by virtue of the stable and efficient cytidine-5′-monophospho-N-acetylneuraminic acid(CMP-NANA) probe and intermediate glutaraldehyde(GA) linker, and the high surface-to-volume ratio of the SiNWs[58]
Wuet al. used sidewall spacer to fabricate polycrystalline silicon NWFET sensors. This NWFET sensor with 3µm nanowires had high sensitivity under low concentrations of biomolecules and sensitivity identical to that of the NWFET with 1.6µm nanowires under moderate concentrations. They also demonstrated that the lower the number of nanowires was, the higher the sensitivity of the NWFET sensor. Both the length and number of nanowires had little effect on LOD[59].
Although silicon nanowire field effect transistors have high detection sensitivity and response speed in the field of biomolecular detection, due to the small size of silicon nanowires, complex preparation process, easy oxidation of the surface, it is difficult to achieve industrialization. In addition, silicon nanowire electronic devices require functional modification of the silicon surface in the process of biomolecular detection, which greatly increases the complexity of detection. Therefore, the development of new material FET biosensors with high performance and simple preparation process has always been the research goal of researchers.
3. Summary and prospective
In recent years, viral zoonoses have caused enormous economic loss, making an urgent need for highly sensitive and accurate virus detection methods. A variety of electrochemical and optical semiconductor biosensors for pathogens of zoonoses have been developed, which are different from traditional detection methods, without virus isolation, culture, and nucleotides amplification.
One of the development trends of semiconductor biosensors is the application of new materials to improve the sensitivity and specificity, such as nanomaterials. As reviewed in this study, graphene, carbon nanotube, and silicon nanowire were widely used in semiconductor biosensors as electron transfer materials, electrochemical impedance materials, field effect transistors and as photon/phonon transfer materials. Several zoonoses including Hepatitis B virus, Influenza virus, COVID-19, Dengue virus had developed semiconductor biosensors using nanomaterials. The results showed that the limit of the detection had been improved, 0.08 pM for Dengue virus (Table 2). The extensive research progress in nanotechnology for nanomaterial exploration and the development of new mechanisms in the future will enable researchers to develop highly sensitive, specific and unobtrusive nanosensors for analyzing zoonoses at an affordable cost.
Another trend for semiconductor biosensors is the development of point-of-care (POC) devices for the detection of zoonoses. POC devices show advantages such as privacy and theft protection, medical recommendations, and time- and cost-intensive laboratory processing without sample pretreatment. However, it puts forward higher requirements on the sensitivity, specificity and other parameters to the development of this kind of semiconductor biosensors. The smart optoelectronic nano system, using precise diagnostics without interferents or loss to choose a real sample source also will be the aim of the researchers.
Besides, innovations like applications of e-nose technologies, software innovations and progress in micro-circuitry design and systems integration are also needed, which is significant interest in methods for the early detection of quality changes in diagnosis of zoonosis. The novel devices mentioned in detecting different biomolecular analytes in a real-time, highly precise, specific, and label-free manner have been validated by numerous research studies. Further improving semiconductor biosensors needs close collaboration between material scientists, chemists, physicists, engineers as well as medical personnel.
Overall, various ideas related to semiconductor biosensor systems are constantly emerging and can improve the semiconductor biosensor performance in many ways, including sensitive, accurate, inexpensive, convenient, multiplexed, fully integrated, and wireless communicable semiconductor biosensor systems for pathogen diagnosis. These will hopefully lead to successful development and commercialization of semiconductor biosensors.
References
[1] S D Judson, P M Rabinowitz. Zoonoses and global epidemics. Curr Opin Infect Dis, 34, 385(2021).
[2] M Ciotti, M Ciccozzi, M Pieri et al. The COVID-19 pandemic: Viral variants and vaccine efficacy. Crit Rev Clin Lab Sci, 59, 66(2022).
[3] A S Bernstein, A W Ando, T Loch-Temzelides et al. The costs and benefits of primary prevention of zoonotic pandemics. Sci Adv, 8, eabl4183(2022).
[4] H Chen, K K Liu, Z Li et al. Point of care testing for infectious diseases. Clin Chim Acta, 493, 138(2019).
[5] S Roychoudhury, A Das, P Sengupta et al. Viral pandemics of the last four decades: Pathophysiology, health impacts and perspectives. Int J Environ Res Public Health, 17, 9411(2020).
[6] The National Health Commission of PRC. China Health Statistics Yearbook 2019. Peking Union Medical College Press, 2020
[7] R Ravina, A Dalal, H R Mohan et al. Detection methods for influenza A H1N1 virus with special reference to biosensors: A review. Biosci Rep, 40, BSR20193852(2020).
[8] J Q Bu, Z W Deng, H Liu et al. Current methods and prospects of coronavirus detection. Talanta, 225, 121977(2021).
[9] G Albertoni, M J Girao, N Schor. Mini review: current molecular methods for the detection and quantification of hepatitis B virus, hepatitis C virus, and human immunodeficiency virus type 1. Int J Infect Dis, 25, 145(2014).
[10] J Kang, A Tahir, H J Wang et al. Applications of nanotechnology in virus detection, tracking, and infection mechanisms. WIREs Nanomed Nanobiotechnol, 13, e1700(2021).
[11] J Wang, Z F Wang. Strengths, weaknesses, opportunities and threats (SWOT) analysis of China’s prevention and control strategy for the COVID-19 epidemic. Int J Environ Res Public Health, 17, 2235(2020).
[12] C E M Coltart, B Lindsey, I Ghinai et al. The Ebola outbreak, 2013-2016: Old lessons for new epidemics. Philos Trans Royal Soc B, 372, 20160297(2017).
[13] N Høiby. Pandemics: Past, present, future: That is like choosing between cholera and plague. APMIS, 129, 352(2021).
[14] H G Davies, C Bowman, S P Luby. Cholera – management and prevention. J Infect, 74, S66(2017).
[15] P Koedrith, T Thasiphu, J I Weon et al. Recent trends in rapid environmental monitoring of pathogens and toxicants: Potential of nanoparticle-based biosensor and applications. Sci World J, 2015, 510982(2015).
[16] Z Li, P Wang. Point-of-care drug of abuse testing in the opioid epidemic. Arch Pathol Lab Med, 144, 1325(2020).
[17] Y Shen, T B Anwar, A Mulchandani. Current status, advances, challenges and perspectives on biosensors for COVID-19 diagnosis in resource-limited settings. Sens Actuat Rep, 3, 100025(2021).
[18] N Bhalla, P Jolly, N Formisano et al. Introduction to biosensors. Essays Biochem, 60, 1(2016).
[19] K S Park. Nucleic acid aptamer-based methods for diagnosis of infections. Biosens Bioelectron, 102, 179(2018).
[20] S M Yoo, S P Lee. Optical biosensors for the detection of pathogenic microorganisms. Trends Biotechnol, 34, 7(2016).
[21] Y Seok, B Batule, M G Kim. Lab-on-paper for all-in-one molecular diagnostics (LAMDA) of zika, dengue, and chikungunya virus from human serum. Biosens Bioelectron, 165, 112400(2020).
[22] S Kutsuna, S Saito, N Ohmagari. Simultaneous diagnosis of dengue virus, Chikungunya virus, and Zika virus infection using a new point-of-care testing (POCT) system based on the loop-mediated isothermal amplification (LAMP) method. J Infect Chemother, 26, 1249(2020).
[23] S Carinelli, M Kühnemund, M Nilsson et al. Yoctomole electrochemical genosensing of Ebola virus cDNA by rolling circle and circle to circle amplification. Biosens Bioelectron, 93, 65(2017).
[24] L Yang, M Li, F Du et al. A novel colorimetric PCR-based biosensor for detection and quantification of hepatitis B virus. Methods Mol Biol, 1571, 357(2017).
[25] H Eun. Sensitive electrochemical biosensor combined with isothermal amplification for point-of-care COVID-19 tests. Biosens Bioelectron, 182, 113168(2021).
[26] E Cadoni, A Manicardi, A Madder. PNA-based microRNA detection methodologies. Molecules, 25, 1296(2020).
[27] R V Dixon, E Skaria, W M Lau et al. Microneedle-based devices for point-of-care infectious disease diagnostics. Acta Pharm Sin B, 11, 2344(2021).
[28] S G Ramakrishnan, B Robert, A Salim et al. Nanotechnology based solutions to combat zoonotic viruses with special attention to SARS, MERS, and COVID 19: Detection, protection and medication. Microb Pathog, 159, 105133(2021).
[29] N Dadina, J Tyson, S Zheng et al. Imaging organelle membranes in live cells at the nanoscale with lipid-based fluorescent probes. Curr Opin Chem Biol, 65, 154(2021).
[30] Elham . Nanomaterial application in bio/sensors for the detection of infectious diseases. Talanta, 230, 122026(2021).
[31] D C Ferrier, K C Honeychurch. Carbon nanotube (CNT)-based biosensors. Biosensors, 11, 486(2021).
[32] M M S Silva, A C M S Dias, B V M Silva et al. Electrochemical detection of dengue virus NS1 protein with a poly(allylamine)/carbon nanotube layered immunoelectrode. J Chem Technol Biotechnol, 90, 194(2015).
[33] M Chen, C J Hou, D Q Huo et al. An ultrasensitive electrochemical DNA biosensor based on a copper oxide nanowires/single-walled carbon nanotubes nanocomposite. Appl Surf Sci, 364, 703(2016).
[34] R L Pinals, F Ledesma, D Yang et al. Rapid SARS-CoV-2 spike protein detection by carbon nanotube-based near-infrared nanosensors. Nano Lett, 21, 2272(2021).
[35] Daniel . A heparin-functionalized carbon nanotube-based affinity biosensor for dengue virus. Biosens Bioelectron, 91, 811(2017).
[36] Y X Fu, V Romay, Y Liu et al. Chemiresistive biosensors based on carbon nanotubes for label-free detection of DNA sequences derived from avian influenza virus H5N1. Sens Actuat B, 249, 691(2017).
[37] W T Shao, M R Shurin, S E Wheeler et al. Rapid detection of SARS-CoV-2 antigens using high-purity semiconducting single-walled carbon nanotube-based field-effect transistors. ACS Appl Mater Interfaces, 13, 10321(2021).
[38] E Vermisoglou, D Panáček, K Jayaramulu et al. Human virus detection with graphene-based materials. Biosens Bioelectron, 166, 112436(2020).
[39] J Lee, K Takemura, E Y Park. Plasmonic nanomaterial-based optical biosensing platforms for virus detection. Sensors, 17, 2332(2017).
[40] Ü Anik, Y Tepeli, M Sayhi et al. Towards the electrochemical diagnostic of influenza virus: Development of a graphene-Au hybrid nanocomposite modified influenza virus biosensor based on neuraminidase activity. Analyst, 143, 150(2017).
[41] S R Joshi, A Sharma, G H Kim et al. Low cost synthesis of reduced graphene oxide using biopolymer for influenza virus sensor. Mater Sci Eng C Mater Biol Appl, 108, 110465(2020).
[42] S Jeong, D M Kim, S Y An et al. Fluorometric detection of influenza viral RNA using graphene oxide. Anal Biochem, 561/562, 66(2018).
[43] X Jin, H Zhang, Y T Li et al. A field effect transistor modified with reduced graphene oxide for immunodetection of Ebola virus. Mikrochim Acta, 186, 223(2019).
[44] A Maity, X Y Sui, B Jin et al. Resonance-frequency modulation for rapid, point-of-care Ebola-glycoprotein diagnosis with a graphene-based field-effect biotransistor. Anal Chem, 90, 14230(2018).
[45] N A S Omar, Y W Fen, J Abdullah et al. Sensitive detection of dengue virus type 2 E-proteins signals using self-assembled monolayers/reduced graphene oxide-PAMAM dendrimer thin film-SPR optical sensor. Sci Rep, 10, 2374(2020).
[46] N A S Omar, Y W Fen, J Abdullah et al. Quantitative and selective surface plasmon resonance response based on a reduced graphene oxide-polyamidoamine nanocomposite for detection of dengue virus E-proteins. Nanomaterials, 10, 569(2020).
[47] P Kanagavalli, M Veerapandian. Opto-electrochemical functionality of Ru(II)-reinforced graphene oxide nanosheets for immunosensing of dengue virus non-structural 1 protein. Biosens Bioelectron, 150, 111878(2020).
[48] J L Fan, L Q Yuan, Q X Liu et al. An ultrasensitive and simple assay for the Hepatitis C virus using a reduced graphene oxide-assisted hybridization chain reaction. Analyst, 144, 3972(2019).
[49] A Valipour, M Roushani. Using silver nanoparticle and thiol graphene quantum dots nanocomposite as a substratum to load antibody for detection of hepatitis C virus core antigen: Electrochemical oxidation of riboflavin was used as redox probe. Biosens Bioelectron, 89, 946(2017).
[50] J Ku, K Chauhan, S H Hwang et al. Enhanced specificity in loop-mediated isothermal amplification with poly(ethylene glycol)-engrafted graphene oxide for detection of viral genes. Biosensors, 12, 661(2022).
[51] G Seo, G Lee, M J Kim et al. Rapid detection of COVID-19 causative virus (SARS-CoV-2) in human nasopharyngeal swab specimens using field-effect transistor-based biosensor. ACS Nano, 14, 5135(2020).
[52] S Afsahi, M B Lerner, J M Goldstein et al. Novel graphene-based biosensor for early detection of Zika virus infection. Biosens Bioelectron, 100, 85(2018).
[53] S Islam, S Shukla, V K Bajpai et al. A smart nanosensor for the detection of human immunodeficiency virus and associated cardiovascular and arthritis diseases using functionalized graphene-based transistors. Biosens Bioelectron, 126, 792(2019).
[54] J L Gogola, G Martins, A Gevaerd et al. Label-free aptasensor for p24-HIV protein detection based on graphene quantum dots as an electrochemical signal amplifier. Anal Chim Acta, 1166, 338548(2021).
[55] M F Abd Muain, K H Cheo, M N Omar et al. Gold nanoparticle-decorated reduced-graphene oxide targeting anti hepatitis B virus core antigen. Bioelectrochemistry, 122, 199(2018).
[56] Q Xiang, J Y Huang, H Y Huang et al. A label-free electrochemical platform for the highly sensitive detection of hepatitis B virus DNA using graphene quantum dots. RSC Adv, 8, 1820(2018).
[57] S K Yong, S K Shen, C W Chiang et al. Silicon nanowire field-effect transistor as label-free detection of hepatitis B virus proteins with opposite net charges. Biosensors, 11, 442(2021).
[58] M Uhm, J M Lee, J Lee et al. Ultrasensitive electrical detection of hemagglutinin for point-of-care detection of influenza virus based on a CMP-NANA probe and top-down processed silicon nanowire field-effect transistors. Sensors, 19, 4502(2019).
[59] C C Wu. Silicon nanowires length and numbers dependence on sensitivity of the field-effect transistor sensor for hepatitis B virus surface antigen detection. Biosensors, 12, 115(2022).
[60] A Wasfi, F Awwad, J G Gelovani et al. COVID-19 detection via silicon nanowire field-effect transistor: Setup and modeling of its function. Nanomaterials, 12, 2638(2022).
[61] B T Gao, A A Rojas Chavez, W I Malkawi et al. Sensitive detection of SARS-CoV-2 spike protein using vertically-oriented silicon nanowire array-based biosensor. Sens Biosensing Res, 36, 100487(2022).