Hongbo Li, Jingyin Xu, Wenyin Wei, En'en Li, Kai Zhang, Hong Li, Yirong Wu, Tianwu Wang, Guangyou Fang. Progress of High Spatiotemporal Resolution Terahertz Scanning Tunneling Microscope for Near-Field Imaging[J]. Laser & Optoelectronics Progress, 2023, 60(18): 1811001

Search by keywords or author
- Laser & Optoelectronics Progress
- Vol. 60, Issue 18, 1811001 (2023)
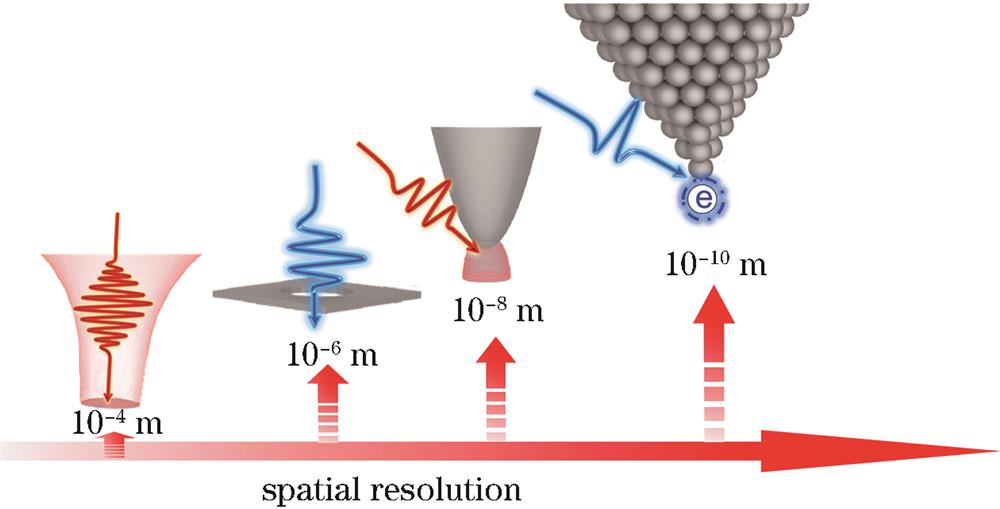
Fig. 1. Development of near-field super-resolution imaging techniques
![Diffusion of Si-adatom dimers[22]. (a) (b) Diffusion of Si-adatom dimers at a temperature of 65 ℃; (c) (d) observation of Si dimer captured by substrate defects at different scales of 70 Å and 90 Å, respectively](/richHtml/lop/2023/60/18/1811001/img_02.jpg)
Fig. 2. Diffusion of Si-adatom dimers[22]. (a) (b) Diffusion of Si-adatom dimers at a temperature of 65 ℃; (c) (d) observation of Si dimer captured by substrate defects at different scales of 70 Å and 90 Å, respectively
![Conceptual diagram of photoconductive-gated STM and junction-mixing STM. (a) Optical path of pump light and probe light illuminating the sample and tip with a certain time delay, utilizing photoconductive bias to control the tunneling current[24]; (b) optical path of two laser beams simultaneously illuminating the sample end with a certain optical path difference, exciting picosecond pulse voltage[26]](/Images/icon/loading.gif)
Fig. 3. Conceptual diagram of photoconductive-gated STM and junction-mixing STM. (a) Optical path of pump light and probe light illuminating the sample and tip with a certain time delay, utilizing photoconductive bias to control the tunneling current[24]; (b) optical path of two laser beams simultaneously illuminating the sample end with a certain optical path difference, exciting picosecond pulse voltage[26]
![Direct coupling optical experiment and its thermal analysis. (a) Experiment operated by Hamers et al.[31]; (b) relationship between chopping frequency and thermal expansion effect[32]](/Images/icon/loading.gif)
Fig. 4. Direct coupling optical experiment and its thermal analysis. (a) Experiment operated by Hamers et al.[31]; (b) relationship between chopping frequency and thermal expansion effect[32]
![Diagram of the SPPX-STM method and its current modulation principle. (a) Experiment operated by Takeuchi et al.[34]; (b) symmetrical current peak generated by autocorrelation scanning laser[34]; (c) case of delayed sinusoidal modulation[34]; (d) case of delayed square wave modulation[35]](/Images/icon/loading.gif)
Fig. 5. Diagram of the SPPX-STM method and its current modulation principle. (a) Experiment operated by Takeuchi et al.[34]; (b) symmetrical current peak generated by autocorrelation scanning laser[34]; (c) case of delayed sinusoidal modulation[34]; (d) case of delayed square wave modulation[35]
![Exploring carrier dynamics in GaAs-PIN junction structures using the SPPX-STM method[72]. (a) Conceptual diagram of heterojunction; (b) energy band diagram; (c) time-resolved carrier diffusion diagram](/Images/icon/loading.gif)
Fig. 6. Exploring carrier dynamics in GaAs-PIN junction structures using the SPPX-STM method[72]. (a) Conceptual diagram of heterojunction; (b) energy band diagram; (c) time-resolved carrier diffusion diagram
![Introduction to the basic characteristics and principles of THz-STM. (a) Tip coupled with THz[6]; (b) I-V curve of the STM tunneling junction under the influence of THz in a biased form[6]; (c) (d) thermal effect of THz[86]; (e) enhancement of field intensity due to the tip antenna effect[82]; (f) Keldysh model considering the transition probability of Volkov states scattered by needle point electronic states into air[85]](/Images/icon/loading.gif)
Fig. 7. Introduction to the basic characteristics and principles of THz-STM. (a) Tip coupled with THz[6]; (b) I-V curve of the STM tunneling junction under the influence of THz in a biased form[6]; (c) (d) thermal effect of THz[86]; (e) enhancement of field intensity due to the tip antenna effect[82]; (f) Keldysh model considering the transition probability of Volkov states scattered by needle point electronic states into air[85]
![Model of tunneling current in THz-STM and influence of polarization and carrier phase. (a) THz response observed for polarization perpendicular to the tip[86]; (b) current responses of THz under various carrier phase conditions[87]; (c) Bardeen model considering the metal surface[83]; (d) THz peak current and rectified current[83]; (e) schematic of THz carrier envelope phase shifter[89]](/Images/icon/loading.gif)
Fig. 8. Model of tunneling current in THz-STM and influence of polarization and carrier phase. (a) THz response observed for polarization perpendicular to the tip[86]; (b) current responses of THz under various carrier phase conditions[87]; (c) Bardeen model considering the metal surface[83]; (d) THz peak current and rectified current[83]; (e) schematic of THz carrier envelope phase shifter[89]
![Source of THz-STM rectification current signal[6]. (a) Transient current pulse of 100-500 fs generated by a THz transient bias voltage of up to 10 V magnitude; (b) transient current pulse rectified by a pre current amplifier and chopping modulation](/Images/icon/loading.gif)
Fig. 9. Source of THz-STM rectification current signal[6]. (a) Transient current pulse of 100-500 fs generated by a THz transient bias voltage of up to 10 V magnitude; (b) transient current pulse rectified by a pre current amplifier and chopping modulation
![THz-STM time resolution capability[86]. (a) THz autocorrelation pulse pairs acting on the STM needle tip; (b) increasing the peak electric field intensity of THz, there is a growth threshold in the tunneling current variation curve caused by THz on Au nanoislands and HOPG samples; (c) increasing the peak electric field intensity of THz results in changes in the full width at half maximum of THz autocorrelation peaks on both samples; (d) (e) amplitude and full width at half maximum of THz autocorrelation peaks on two samples as a function of THz electric field intensity](/Images/icon/loading.gif)
Fig. 10. THz-STM time resolution capability[86]. (a) THz autocorrelation pulse pairs acting on the STM needle tip; (b) increasing the peak electric field intensity of THz, there is a growth threshold in the tunneling current variation curve caused by THz on Au nanoislands and HOPG samples; (c) increasing the peak electric field intensity of THz results in changes in the full width at half maximum of THz autocorrelation peaks on both samples; (d) (e) amplitude and full width at half maximum of THz autocorrelation peaks on two samples as a function of THz electric field intensity
![Steady state imaging of THz-STM on various samples. (a) Nanoresolution imaging of Au nanoislands on HOPG[6]; (b) THz constant height mode imaging of armchair graphene strips grown on Au111[60]; (c) imaging of THz-STM on Si111 is shown in the left image with THz driven scanning (40 nm×40 nm), the built-in image shows amplification of a specific area (8.5 nm×8.5 nm), and the right image shows the height difference between STM imaging and THz driven imaging within the red line range[58]; (d) imaging of THz-STM using phase-locked amplification mode on metal Cu111[83]](/Images/icon/loading.gif)
Fig. 11. Steady state imaging of THz-STM on various samples. (a) Nanoresolution imaging of Au nanoislands on HOPG[6]; (b) THz constant height mode imaging of armchair graphene strips grown on Au111[60]; (c) imaging of THz-STM on Si111 is shown in the left image with THz driven scanning (40 nm×40 nm), the built-in image shows amplification of a specific area (8.5 nm×8.5 nm), and the right image shows the height difference between STM imaging and THz driven imaging within the red line range[58]; (d) imaging of THz-STM using phase-locked amplification mode on metal Cu111[83]
![Field-induced fluorescence effect in THz-STM[94]. (a) Morphology of Au islands on Ag111; (b) fluorescence differences at the ends and sides of Au islands; (c) imaging for Au islands through fluorescence differences](/Images/icon/loading.gif)
Fig. 12. Field-induced fluorescence effect in THz-STM[94]. (a) Morphology of Au islands on Ag111; (b) fluorescence differences at the ends and sides of Au islands; (c) imaging for Au islands through fluorescence differences
![Single-point pump-probe detection experiment in THz-STM. (a) SEM image of InAs quantum dots grown on Au111[6]; (b) THz detection of the charging and discharging process of InAs quantum dots under 800 nm infrared light pumping[6]; (c) STM scanning of 2H-MoTe2 bulk material[62]; (d) experiment involving 1030-nm pumping and THz detection[62]; (e) schematic of the pumping and detection process[62]; (f) pumping and detection curves under varying pump intensities[62]](/Images/icon/loading.gif)
Fig. 13. Single-point pump-probe detection experiment in THz-STM. (a) SEM image of InAs quantum dots grown on Au111[6]; (b) THz detection of the charging and discharging process of InAs quantum dots under 800 nm infrared light pumping[6]; (c) STM scanning of 2H-MoTe2 bulk material[62]; (d) experiment involving 1030-nm pumping and THz detection[62]; (e) schematic of the pumping and detection process[62]; (f) pumping and detection curves under varying pump intensities[62]
![High spatio-temporal resolution scanning in THz-STM. (a) Discharge process of InAs quantum dots on Au[6]; (b) longitudinal diffusion process of hot carriers on Au in C60[95]](/Images/icon/loading.gif)
Fig. 14. High spatio-temporal resolution scanning in THz-STM. (a) Discharge process of InAs quantum dots on Au[6]; (b) longitudinal diffusion process of hot carriers on Au in C60[95]
|
Table 1. Development of time-resolved STM
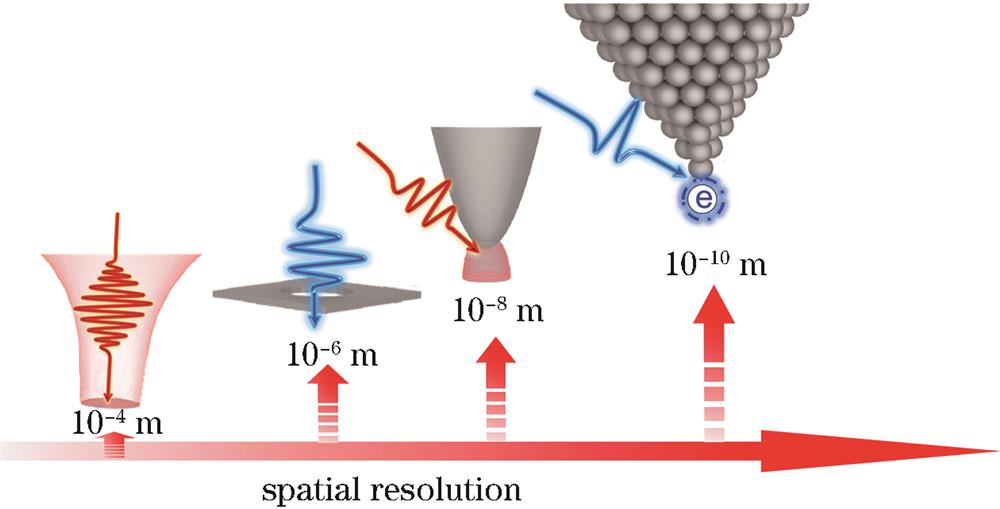
Set citation alerts for the article
Please enter your email address