
- Chinese Optics Letters
- Vol. 19, Issue 9, 091204 (2021)
Abstract
1. Introduction
Optical fiber sensors have been developed rapidly in the last few decades, and more and more applications are emerging. The main advantages of optical fiber sensors include high sensitivity, light weight, small volume, anti-corrosion, immunity to electro-magnetic interference, distributed and remote sensing capability, and the suitability for extreme environment monitoring such as high temperature. High temperature sensing has important applications in energy, iron-steel, aero-engine, defense, and military industries, which has induced intensive research interests in recent years[
Optical fiber high temperature sensors can be realized by different types of optical fiber structures and configurations and constructed in different types of optical fibers. The most popular fiber structures used for high temperature sensing are fiber Bragg grating (FBG)[
FBG-based optical fiber sensors are simple, compact, highly flexible, and convenient in use, and can be easily multiplexed in a series along a single optical fiber, thus achieving simultaneous multi-point sensing in a flexible manner. However, they usually have limited sensitivity (0.01 nm/°C). The FBG is essentially a simple wavelength mirror or filter, usually fabricated by exposing the optical fiber to ultraviolet (UV) laser light using the interferometric method or the phase mask technique, which causes a periodic modulation of the refractive index of the optical fiber, arising from the fiber’s inherent photosensitivity[
Sign up for Chinese Optics Letters TOC. Get the latest issue of Chinese Optics Letters delivered right to you!Sign up now
LPFG-based sensors exhibit higher sensitivity to external perturbations than that of FBGs. LPFGs exhibit periodic structures that couple light from the guided core mode to the cladding mode at resonant wavelengths satisfying the phase matching condition. The transmission spectra of LPFGs consist of a series of attenuation bands centered at the resonant wavelengths, which are sensitive to grating period, grating length, and environmental parameters such as strain, bend, and temperature. LPFG-based sensors have a relatively large device size (on the order of centimeters) and a wide 3 dB bandwidth, which leads to a low measurement resolution. Moreover, the LPFG is sensitive to an external refractive index and bending, thus producing cross sensitivity.
Optical fiber interferometric high temperature sensors are featured with high sensitivity, without age decaying or structure erasing problems faced by FBGs. Different types of interferometer configurations have been developed for high temperature sensors, such as the Mach–Zehnder interferometer (MZI)[
Besides the frequently used conventional single-mode fiber (SMF), multimode fiber (MMF), photonics crystal fiber (PCF) or microstructured fiber, microfiber, and other specialty optical fibers are also employed for high temperature measurement[
In the past two decades, the femtosecond laser has become a powerful and flexible tool for optical fiber high temperature sensor fabrication. It can be used for a variety of materials, with ultrashort processing time, high processing precision, and small heat affected zone[
In this paper, we will review the recent progress of femtosecond laser fabricated optical fiber high temperature sensors. The main interests lie in the sensor device based on femtosecond laser fabricated fiber gratings and various types of fiber in-line interferometers in silica fibers and sapphire fibers.
2. Femtosecond Laser Fabricated Fiber Gratings for High Temperature Sensing
FBGs are usually written by use of either the phase mask method or point-by-point technique[
The UV laser is typically used to write FBGs, in which the refractive index modulation produced depends on the photosensitivity of the fiber materials and usually has poor sustainability at high temperatures. The femtosecond laser can produce the refractive index modulation in almost any kind of transparent material[
Figure 1 demonstrates the structures of type I and type II FBGs written by the femtosecond laser. Type II FBGs have a structure with a permanent refractive index change, are much more stable under high temperatures than type I FBGs, which refers to the grating formed under normal laser irradiation intensity, and can be erased at a relatively low temperature.
Figure 1.Optical microscope images of FBGs inscribed by IR fs lasers[
One of the limitation factors for further enhancing the thermal stability of the FBG is the residual stress that exists in the optical fiber fabrication process, caused by the mechanical property and thermal expansion coefficient differences between the fiber core and cladding[
Figure 2 shows a long-term thermal stability test on type II gratings. It can be observed that the FBGs written in the fibers with pre-annealing treatment of 1100°C have enhanced thermal stability, almost unaffected by the thermal exposure to temperatures up to 1200°C.
Figure 2.Change in the reflectivity of the type II-IR FBGs inscribed in normal and pre-annealed fibers over a 1300 min period at an annealing temperature of 1200°C[
One of the problems of the pre-annealing treatment of fiber is that the fiber becomes brittle, which creates difficulty in the grating fabrication. Such a problem can be alleviated to some extent by introducing compressive residual stress in FBGs through high temperature annealing followed by a rapid air quenching treatment, which can build up compressive residual stresses in the optical fiber, similar to the annealing of the glass[
Figures 3(a) and 3(b) demonstrate the evolution of the grating reflectivity and the resonance wavelength, respectively. It can be observed that the pre-stressed gratings exhibit clearly enhanced thermal stability, which is almost unaffected by thermal exposure at temperatures up to 1200°C, and there is only a slight fluctuation of the grating strength during the 26 h test.
Figure 3.Evolutions of (a) the reflection and (b) the resonant wavelength of the pre-stressed FBGs (fabricated using 550 µJ pulse energy) over 26 h at the annealing temperature of 1200°C.
In a recent investigation, both doped silica fiber and pure silica fiber were tested at high temperature[
FBGs are also written by femtosecond lasers in the MMF[
Recently, a linear-cavity fiber laser based on an FBG fabricated by a femtosecond laser has been developed and tested for its high temperature characteristics, such a fiber laser can operate stably at 1000°C with a temperature sensitivity of 15.9 pm/°C in the range of 300–1000°C[
The femtosecond laser inscribed LPFGs are reported in Refs. [62,63] with a relatively large temperature sensitivity of more than 100 pm/°C.
3. Optical Fiber In-Line Interferometers for High Temperature Sensing
Although many optical fiber interferometers can perform high temperature sensing, fiber in-line interferometers have the advantages of compact size, flexible arrangement, and convenient operation. By use of an efficient femtosecond laser micromachining technique, various types of fiber in-line interferometers have been developed and used for high temperature sensing.
Figure 4 shows the schematic examples of the femtosecond laser fabricated FPI, MZI, and MI suitable for high temperature monitoring. In Fig. 4(a), part of the fiber cladding and a small section of the whole fiber core are removed by femtosecond laser micromachining, and an open air-cavity is created. Part of the incident light traveling in the fiber core is reflected by the first end face of the air cavity, and the rest keeps traveling in the air cavity and is reflected by the second end face of the air cavity before returning to the fiber core at the first end face position, thus forming an FPI.
Figure 4.Schematics of the femtosecond laser fabricated fiber in-line interferometers. (a) FPI, (b) MZI, and (c) MI.
Figure 4(b) is similar to Fig. 4(a), but only a small section of half of the fiber core is removed, which allows that part of the incident light to pass through the open air cavity, while the rest remains traveling in the fiber core; both are recombined at the air-cavity end and form an MZI.
In Fig. 4(c), a section of the optical fiber, including half of the fiber core and cladding, is removed. The incident light traveling in the fiber core is firstly divided into two beams and reflected by the two fiber end faces, respectively, before recombining in the fiber core at the first fiber end face position and forming an MI.
The fiber in-line interferometers can be constructed by using different fibers, fiber structures, and configurations.
Similar to the configuration shown in Fig. 4(a), a fiber in-line FPI can be constructed by drilling a micro-channel crossing the fiber core using femtosecond laser [
Recently, in-fiber reflection mirrors have been inscribed in SMFs, NCFs, and MMFs by a femtosecond laser, which can be flexibly arranged to form cascaded FPIs in a series or a parallel structure with precisely controlled FP cavity length[
As shown in Fig. 4(b), by removing part of the fiber core and cladding using femtosecond laser micromachining, an MZI is formed and used for high temperature monitoring up to 1100°C[
In Ref. [78], a fiber in-line MI similar to that displayed in Fig. 4(c) is constructed, with the help of femtosecond laser micromachining. The device can be used for high temperature sensing up to 1000°C, with the temperature sensitivity of 14.72 pm/°C. In Ref. [28], an inclined narrow slit inside the SMF crossing the fiber core is created by using femtosecond laser micromachining, and the narrow slit plays the role of an in-fiber beam splitter. The optical fiber in-line MI fabricated is found to have good high temperature sustainability up to 1000°C. In Ref. [79], a fiber in-line MI high temperature sensor is constructed by using a femtosecond laser to cut a 45° fiber end.
4. Femtosecond Laser Fabricated FBGs in Sapphire Fibers for High Temperature Sensing
Since the glass transition temperature of the silica is around 1050°C, the long-term stability of the SMF and microstructure fiber is typically below 1200°C. For the high temperature optical fiber sensing of greater than 1200°C, the sapphire fiber has to be utilized[
5. Conclusion
Various types of optical fiber sensors based on FBGs, LPFGs, and fiber in-line interferometers have been fabricated in SMFs, MMFs, and microstructured fibers by use of a femtosecond laser for the high temperature sensing up to 1200°C and, for a long time, limited by the glass transition temperature of silica. For the higher temperature sensing up to 2000°C, the femtosecond laser is even an indispensible tool for writing FBGs in sapphire fibers.
Compared with other techniques for optical fiber high temperature sensor construction, femtosecond laser irradiation is featured with wide material processing suitability, rapid processing speed, high processing precision, and small heat affected zone, which ensures good processing quality and flexible fabrication capability. It is expected that the femtosecond laser will play a more and more important role in fabricating optical fiber high temperature sensors suitable for extreme environment monitoring.
References
[1] X. N. Jiang, K. Kim, S. J. Zhang, J. Johnson, G. Salazar. High-temperature piezoelectric sensing. Sensors, 14, 144(2014).
[2] M. R. Islam, M. M. Ali, M. H. Lai, K. S. Lim, H. Ahmad. Chronology of Fabry–Perot interferometer fiber-optic sensors and their applications: a review. Sensors, 14, 7451(2014).
[3] C. R. Liao, D. N. Wang. Review of femtosecond laser fabricated fiber Bragg gratings for high temperature sensing. Photon. Sens., 3, 97(2013).
[4] S. J. Mihailov, D. Grobnic, C. Hnatovsky, R. B. Walker, P. Lu, D. Coulas, H. Ding. Extreme environment sensing using femtosecond laser-inscribed fiber Bragg gratings. Sensors, 17, 2909(2017).
[5] J. K. Sahota, G. Neena, D. D. Dhawan. Fiber Bragg grating sensors for monitoring of physical parameters: a comprehensive review. Opt. Eng., 59, 060901(2020).
[6] G. Brambilla, H. Rutt. Fiber Bragg gratings with enhanced thermal stability. Appl. Phys. Lett., 80, 3259(2002).
[7] A. Martinez, I. Y. Khrushchev, I. Bennion. Thermal properties of fibre Bragg gratings inscribed point-by-point by infrared femtosecond laser. Electron. Lett., 41, 176(2005).
[8] D. Grobnic, C. W. Smelser, S. J. Mihailov, R. B. Walker. Long-term thermal stability tests at 1000°C of silica fibre Bragg gratings made with ultrafast laser radiation. Meas. Sci. Technol., 17, 1009(2006).
[9] B. Zhang, M. Kahrizi. High-temperature resistance fiber Bragg grating temperature sensor fabrication. IEEE Sens. J., 7, 586(2007).
[10] J. Canning, M. Stevenson, S. Bandyopadhyay, K. Cook. Extreme silica optical fibre gratings. Sensors, 8, 6448(2008).
[11] H. Chikn-Bled, K. Chan, A. Gonzalez-Vila, B. Lasri, C. Caucheteur. Bahavior of femtosecond laser induced eccentric fiber Bragg gratings at very high temperatures. Opt. Lett., 41, 4048(2016).
[12] Y. H. Li, C. R. Liao, D. N. Wang, T. Sun, K. T. V. Grattan. Study of spectral and annealing properties of fiber Bragg gratings written in H2-free and H2-loaded fibers by use of femtosecond laser pulses. Opt. Express, 16, 21239(2008).
[13] Y. H. Li, M. W. Yang, D. N. Wang, T. Sun, K. T. V. Grattan. Fiber Bragg gratings with enhanced thermal stability by residual stress relaxation. Opt. Express, 17, 19785(2009).
[14] Y. H. Li, M. Yang, C. R. Liao, D. N. Wang, J. Lu, P. X. Lu. “Prestressed fiber Bragg grating with high temperature stability. IEEE J. Lightwave Technol., 29, 1555(2011).
[15] G. Rego, O. Okhomikov, E. Dianov, V. Sulimov. High-temperature stability of long-period fiber gratings produced using an electric arc. IEEE J. Lightwave Technol., 19, 1574(2001).
[16] G. Humbert, A. Malki, S. Fevrier, P. Roy, D. Pagnoux. Characterizations at high temperatures of long-period gratings written in germanium-free air-silica microstructure fiber. Opt. Lett., 29, 38(2004).
[17] Y. Zhu, P. Shum, H. Bay, M. Yan, X. Yu, J. Hu, J. Hao, C. Lu. Strain-insensitive and high-temperature long-period gratings inscribed in photonic crystal fiber. Opt. Lett., 30, 367(2005).
[18] M. Park, S. Lee, W. Ha, D. K. Kim, W. Shin, I. B. Sohn, K. Oh. Ultracompact intrinsic micro air-cavity fiber Mach–Zehnder interferometer. IEEE Photon. Technol. Lett, 21, 1027(2009).
[19] Y. Wang, Y. Li, C. Liao, D. N. Wang, M. Yang, P. Lu. High-temperature sensing using miniaturized fiber in-line Mach–Zehnder interferometer. IEEE Photon. Technol. Lett, 22, 39(2010).
[20] L. Jiang, J. Yang, S. Wang, B. Li, M. Wang. Fiber Mach–Zehnder interferometer based on microcavities for high-temperature sensing with high sensitivity. Opt. Lett., 36, 3753(2011).
[21] T. Y. Hu, Y. Wang, C. R. Liao, D. N. Wang. Miniaturized fiber in-line Mach–Zehnder interferometer based on inner air-cavity for high-temperature sensing. Opt. Lett., 37, 5082(2012).
[22] H. Y. Choi, K. S. Park, S. J. Park, U.-C. Paek, B. H. Lee, E. S. Choi. Miniature fiber-optic high temperature sensor based on a hybrid structured Fabry–Perot interferometer. Opt. Lett., 33, 2455(2008).
[23] T. Zhu, T. Ke, Y. Rao, K. S. Chiang. Fabry–Perot fiber tip for high temperature measurement. Opt. Commun., 283, 3683(2010).
[24] W. Ding, Y. Jiang, R. Gao, Y. Liu. High-temperature fiber-optic Fabry–Perot interferometeric sensors. Rev. Sci. Instrum., 86, 055001(2015).
[25] D. Lee, M. Yang, C. Huang, J. Dai. Optical fiber high-temperature sensor based on dielectric films extrinsic Fabry–Perot cavity. IEEE Photon. Technol. Lett, 26, 2107(2014).
[26] L. Xu, L. Jiang, S. Wang, B. Li, Y. Lu. High-temperature sensor based on an abrupt-taper Michelson interferometer in single-mode fiber. Appl. Opt., 52, 2038(2013).
[27] H. Cao, X. Shu. Miniature all-fiber high temperature sensor based on Michelson interferometer formed with a novel core-mismatching fiber joint. IEEE Sens. J., 17, 3341(2017).
[28] Y. Liu, D. N. Wang. Fiber in-line Michelson interferometer based on inclined narrow slit crossing the fiber core. IEEE Photon. Technol. Lett, 30, 293(2018).
[29] D. Monzón-Hernández, V. P. Minkovich, J. Villatoro. High-temperature sensing with tapers made of microstructured optical fiber. IEEE Photon. Technol. Lett, 18, 511(2006).
[30] L. V. Nguyen, D. Hwang, S. Moon, D. S. Moon, Y. Chung. High temperature fiber sensor with high sensitivity based on core diameter mismatch. Opt. Express, 16, 11369(2008).
[31] G. Coviello, V. Finazzi, J. Vilatoro, V. Pruneri. Thermally stabilized PCF-based sensor for temperature measurements up to 1000°C. Opt. Express, 17, 21551(2009).
[32] A. V. Newkirk, E. Antonio-Lopez, G. Salceda-Delgado, R. Amezcua-Currea, A. Schulzgen. Optimization of multicore for high-temperature sensing. Opt. Lett., 39, 4812(2014).
[33] S. C. Warren-Smith, L. V. Nguyen, C. Lang, H. Ebendorff-Heidepriem, T. M. Monro. Temperature sensing up to 1300°C using suspended-core microstructured optical fibers. Opt. Express, 24, 3714(2016).
[34] A. Othonos, K. Kalli. Fiber Bragg Gratings(1999).
[35] K. O. Hill, G. Meltz. Fiber Bragg grating technology fundamentals and overview. J. Lightwave Technol., 15, 1263(1997).
[36] G. Brambilla. High temperature fibre Bragg grating thermometer. Electron. Lett., 38, 954(2002).
[37] D. Grobnic, S. J. Mihailov, C. W. Smelser, H. Ding. Sapphire fiber Bragg grating sensor made using femtosecond laser radiation for ultrahigh temperature applications. IEEE Photon. Technol. Lett, 16, 2505(2004).
[38] Y. Zhu, Z. Huang, F. Shen, A. Wang. Sapphire-fiber-based white-light interferometric sensor for high-temperature measurements. Opt. Lett., 30, 711(2005).
[39] M. Busch, W. Ecke, I. Latka, D. Fischer, R. Wilsch, H. Bartelt. Inscription and characterization of Bragg gratings in single-crystal sapphire optical fibres for high-temperature sensor applications. Meas. Sci. Technol, 20, 115301(2009).
[40] J. Wang, B. Dong, E. Lally, J. Gong, M. Han, A. Wang. Multiplexed high temperature sensing with sapphire fiber air gap-based extrinsic Fabry–Perot interferometers. Opt. Lett., 35, 619(2010).
[41] S. J. Mihailov, D. Grobnic, C. W. Smelser. High-temperature multiparameter sensor based on sapphire fiber Bragg gratings. Opt. Lett., 35, 2810(2010).
[42] T. Elsmann, T. Habisreuther, A. Garf, M. Rothhardt, H. Bartelt. Inscription of first-order sapphire Bragg gratings using 400 nm femtosecond laser radiation. Opt. Express, 21, 4591(2013).
[43] T. Habisreuther, T. Elsmann, Z. Pan, A. Garf, M. Rothhardt, R. Wilsch, M. Schmidt. Sapphire fiber Bragg gratings for high temperature and dynamic temperature diagnostics. Appl. Thermal Eng., 91, 860(2015).
[44] X. Xu, J. He, C. Liao, K. Yang, K. Guo, C. Li, Y. Zhang, Z. Ouyang, Y. Wang. Sapphire fiber Bragg gratings inscribed with a femtosecond laser line-by-line scanning technique. Opt. Lett., 43, 4562(2018).
[45] C. Chen, X. Y. Zhang, Y. S. Yu, W. H. Wei, Q. Guo, L. Qin, Y. Q. Ning, L. J. Wang, H. B. Sun. “Femtosecond laser-inscribed high-order Bragg gratings in large-diameter sapphire fibers for high-temperature and strain sensing. IEEE J. Lightwave Technol., 36, 3302(2018).
[46] S. Jeon, V. Malyarchuk, J. A. Rogers, G. P. Wiederrecht. Fabricating three dimensional nanostructures using two photon lithography in a single exposure step. Opt. Express, 14, 2300(2006).
[47] B. N. Chichkov, C. Momma, S. Nolte, F. V. Alvensleben, A. Tunnermann. Femtosecond, picosecond and nanosecond laser ablation of solids. Appl. Phys. A, 63, 109(1996).
[48] S. Maruo, K. Ikuta, H. Korogi. Submicron manipulation tools driven by light in a liquid. Appl. Phys. Lett., 82, 133(2003).
[49] M. D. Perry, B. C. Strart, P. S. Banks, M. D. Feit, V. Yanovsky, A. M. Rubenchik. Ultrafast-pulse laser machining of dielectric materials. J. Appl. Phys., 85, 6803(1999).
[50] N. Abdukerim, D. Grobnic, C. Hnatovsky, S. J. Mihailov. High-temperature-stable fiber Bragg gratings with ultra strong cladding modes written using the phase mask technique and an infrared femtosecond laser. Opt. Lett., 45, 443(2020).
[51] Y. Zhu, H. Mei, T. Zhu, J. Zhang, S. Z. Yin. Dual-wavelength FBG inscribed by femtosecond laser for simultaneous measurement of high temperature and strain. Chin. Opt. Lett., 7, 675(2009).
[52] K. Itoh, W. Watanable, S. Nolte, C. B. Schaffer. Ultrafast processes for bulk modification of transparent materials. MRS Bull., 31, 620(2006).
[53] C. W. Smelser, S. J. Mihailov, D. Grobnic. Formation of Type I-IR and Type II-IR laser and a phase mask. Opt. Express, 13, 5377(2005).
[54] U. C. Paek, C. R. Kurkjian. Calculation of cooling rate and induced stresses in drawing of optical fibers. J. Am. Ceram. Soc., 58, 330(1975).
[55] S. C. Warren-Smith, E. P. Schartner, L. V. Nguyen, D. E. Otten, Z. Yu, D. G. Lancaster, H. Ebendorff-Heidepriem. Stability of grating-based optical fiber sensors at high temperature. IEEE Sens. J., 19, 2978(2019).
[56] Z. Yong, C. Zhan, J. Lee, S. Z. Yin. Multiple parameter vector bending and high-temperature sensors based on asymmetric multimode fiber Bragg gratings inscribed by an infrared femtosecond laser. Opt. Lett., 31, 1794(2006).
[57] T. T. Yang, X. G. Qiao, Q. Z. Rong, W. J. Bao. Fiber Bragg gratings inscriptions in multimode fiber using 800 nm femtosecond laser for high-temperature strain measurement. Opt. Laser Technol., 93, 138(2017).
[58] C. M. Jewart, Q. Q. Wang, J. Canning, D. Grobnic, S. J. Mihailov, K. P. Chen. Ultrafast femtosecond-laser-induced fiber Bragg gratings in air-hole microstructured fibers for high-temperature pressure sensing. Opt. Lett., 35, 1443(2010).
[59] R. Z. Wang, J. H. Si, T. Chen, L. H. Yan, H. J. Cao, X. Pham, X. Hou. Fabrication of high-temperature tilted fiber Bragg gratings using a femtosecond laser. Opt. Express, 25, 23684(2017).
[60] Y. P. Wang, Z. Li, S. Liu, C. Fu, Z. Li, Z. Zhang, Y. Wang, J. He, Z. Bai, C. R. Liao. Parallel-integrated fiber Bragg gratings inscribed by femtosecond laser point-by-point technology. IEEE J. Lightwave Technol., 37, 2185(2019).
[61] F. Huang, T. Chen, J. Si, X. Pham, X. Hou. Fiber laser based on a fiber Bragg grating and its application in high-temperature sensing. Opt. Commun., 452, 233(2019).
[62] Y. Liu, S. L. Qu. Femtosecond laser pulses induced ultra-long-period fiber gratings for simultaneous measurement of high temperature and refractive index. Optik, 124, 1303(2013).
[63] X. R. Dong, Z. Xie, Y. X. Song, Y. Kai, D. K. Chu, J. A. Duan. High temperature-sensitivity sensor based on long period fiber grating inscribed with femtosecond laser transversal-scanning method. Chin. Opt. Lett., 15, 090602(2017).
[64] Y. J. Rao, M. Deng, D. W. Duan, X. C. Yang, T. Zhu, G. H. Cheng. Micro Fabry–Perot interferometers in silica fibers machined by femtosecond laser. Opt. Express, 15, 14123(2007).
[65] C. Zhen, L. Yuan, G. Hefferman, T. Wei. Ultraweak intrinsic Fabry–Perot cavity array for distributed sensing. Opt. Lett., 40, 320(2015).
[66] T. Wei, Y. Han, H. L. Tsai, H. Xiao. Miniaturized fiber inline Fabry–Perot interferometer fabricated with a femtosecond laser. Opt. Lett., 33, 536(2008).
[67] Y. Liu, S. L. Qu, Y. Li. Single microchannel high-temperature fiber sensor by femtosecond laser-induced water breakdown. Opt. Lett., 38, 335(2013).
[68] P. C. Chen, X. W. Shu. Refractive-index-modified-dot Fabry–Perot fiber probe fabricated by femtosecond laser for high-temperature sensing. Opt. Express, 26, 5292(2018).
[69] W. Y. Ma, Y. Jiang, H. C. Gao. Miniature all-fiber extrinsic Fabry–Perot interferometric sensor for high pressure sensing under high-temperature conditions. Meas. Sci. Technol., 30, 025104(2019).
[70] J. Deng, D. N. Wang. Construction of cascaded Fabry–Perot interferometers by four in-fiber mirrors for high-temperature sensing. Opt. Lett., 44, 1289(2019).
[71] Wang Q. H., Zhang H., D. N. Wang. Cascaded multiple Fabry–Perot interferometers fabricated in no-core fiber with a waveguide for high-temperature sensing. Opt. Lett., 44, 5145(2019).
[72] X. L. Cui, H. Zhang, D. N. Wang. Parallel structured optical fiber in-line Fabry–Perot interferometers for high temperature sensing. Opt. Lett., 45, 726(2020).
[73] Y. D. Niu, D. N. Wang, Q. H. Wang, Z. K. Wang, S. Zhang. Cascaded multiple Fabry–Perot interferometers fabricated in multimode fiber with a waveguide. Opt. Fiber Technol., 58, 102306(2020).
[74] H. Zhang, D. N. Wang, B. M. A. Rahman. Parallel structured fiber in-line multiple Fabry–Perot cavities for high temperature sensing. Sens. Actuators A, 313, 112214(2020).
[75] M. Wang, Y. Yang, S. Huang, J. Wu, K. Zhao, Y. Li, Z. Peng, R. Zou, H. Lan, P. R. Ohodnicki, P. Lu, M. P. Buric, B. Liu, Q. Yu, K. P. Chan. Multiplexable high-temperature stable and low-loss intrinsic Fabry–Perot in-fiber sensors through nanograting engineering. Opt. Express, 28, 20225(2020).
[76] H. Gong, D. N. Wang, B. Xu, K. Ni, H. Liu, C. L. Zhao. Miniature and robust optical fiber in-line Mach–Zehnder interferometer based on a hollow ellipsoid. Opt. Lett., 40, 3516(2015).
[77] C. R. Liao, H. F. Chen, D. N. Wang. Ultra compact optical fiber sensor for refractive index and high temperature measurement. IEEE J. Lightwave Technol., 32, 2531(2014).
[78] L. Yuan, T. Wei, Q. Han, H. Z. Wang, J. Huang, L. Jiang, H. Xiao. Fiber inline Michelson interferometer fabricated by a femtosecond laser. Opt. Lett., 37, 4489(2012).
[79] Y. Q. Yu, W. Zhou, J. Ma, S. C. Ruan. High-temperature sensor based on 45° tilted fiber end fabricated by femtosecond laser. IEEE Photon. Technol. Lett, 28, 653(2016).
[80] T. Elsmann, A. Lorenz, N. S. Yazd, T. Habisreuther, J. Dellith, A. Schwuchow, J. Bierlich, K. Schusteret, M. Rothhardt, L. Kido, H. Bartelt. High temperature sensing with fiber Bragg gratings in sapphire-derived all-glass optical fibers. Opt. Express, 22, 26825(2014).
[81] S. Yang, D. Homa, P. Gary, G. Pickrell, A. Wang. Fiber Bragg grating fabricated in micro-single-crystal sapphire fiber. Opt. Lett., 43, 62(2018).
[82] T. Habisreuther, T. Elsmann, Z. W. Pan, A. Graf, R. Willsch, M. A. Schmidt. Sapphire fiber Bragg gratings for high temperature and dynamic temperature diagnostics. Appl. Thermal Eng., 91, 860(2015).
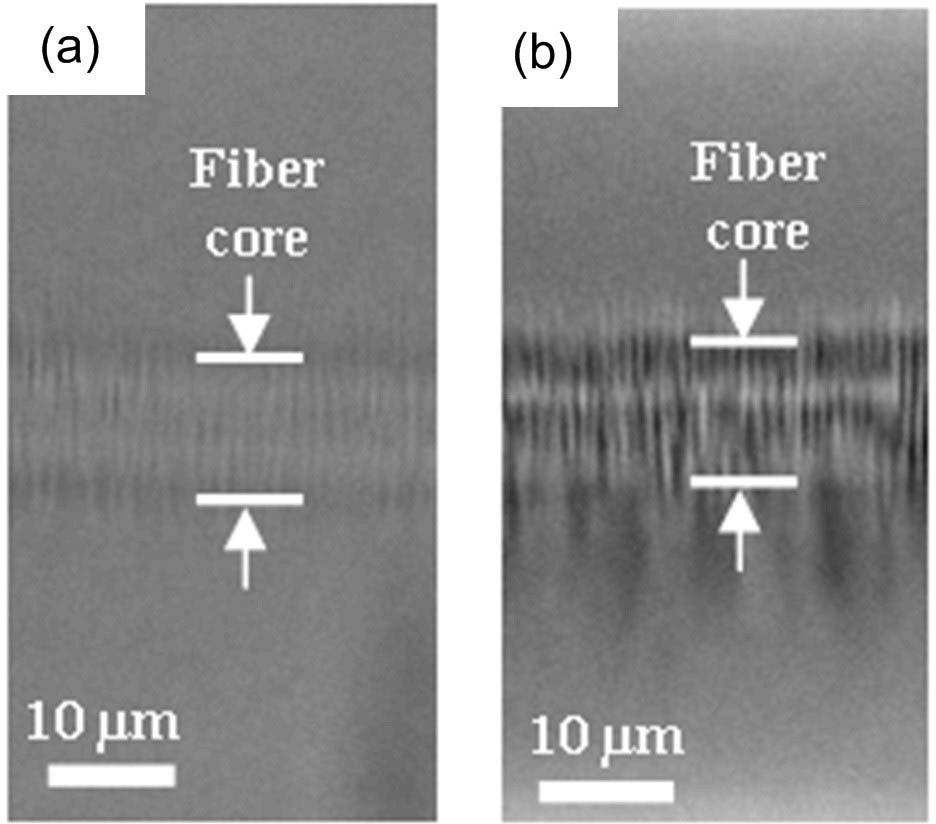
Set citation alerts for the article
Please enter your email address