
- Journal of Inorganic Materials
- Vol. 36, Issue 5, 479 (2021)
Abstract
Keywords
With the enhancement of people's awareness of energy conservation and environmental protection, electrochromic devices (ECDs), used in buildings and vehicles have attracted increasing attention in recent years[
Some metrics are important factors affecting the performance of all-solid-state ECDs, such as optical contrast, cycle stability, especially sputtering efficiency and switching speed[
Taking the above factors into consideration, LiAlOx/ Ta2O5/LiAlOx (ATA) sandwich structured electrolyte with LiAlOx supplying enough lithium ions and Ta2O5 serving as ion transport layer is requisite for optimizing the properties of the novel structured ECDs. The electron- blocking behavior, high ionic conductivity and chemical stability of Ta2O5 makes it an ideal ion transport layer in ECDs. Meanwhile, LiAlOx holds both high optical transmittance and ionic conductivity. Due to the difference of refractive index between LiAlOx and Ta2O5, the sandwich structure electrolyte with alternating high and low refractive index can be designed, which is expected to improve the transmittance using the anti-reflection principle[
In this study, a seven-layer all-solid-state ECD (ITO/NiO/LiAlOx/Ta2O5/LiAlOx/WO3/ITO) incorporated with ATA sandwich structured electrolyte of high sputtering efficiency, high optical transmittance and satisfactory coloration efficiency was designed. Due to effective lithium ions immigration channels provided by ATA sandwich structured electrolyte, the ECD hires remarkably improved switching speed and optimized optical transmittance. Moreover, the sputtering efficiency of ECDs was greatly improved by means of DC continuous magnetron sputtering using metal or alloy targets. The ATA based all-solid-state ECDs by optical design bring a further insight into the mass production and marketization of high-performance ECDs.
1 Experimental
1.1 Materials and synthesis
NiO film and LiAlOx/Ta2O5/LiAlOx sandwich structured electrolyte were deposited on 20 mm×20 mm indium tin oxide (ITO) glass substrates in sequence via reactive DC magnetron sputtering. LiAlOx film was conducted in the flow of Ar/O2 (40 sccm/10 sccm). The sputtering used Li-Al alloy target (99.9%, diameter of 10.16 cm) with 200 W DC power for 45 min at room temperature (RT). Then the middle layer of Ta2O5 was deposited at RT using Ta metal target (99.9%, diameter of 10.16 cm). The sputtering was performed under the condition of 200 W DC power and Ar/O2 ratio of 45:5. For the next step, an amorphous WOx film was deposited in quick succession. Finally, a layer of ITO film was deposited on top of it acting as a top electrode. The detailed deposition parameters of each layer was simulated for max optimization of optical performance in Table 1.
Layer | Target | Pressure/Pa | QAr : QO2 | Power/W | Thickness/nm |
---|---|---|---|---|---|
LiAlOxa | Li-Al | 2.0 | 40 : 10 | 200 | ~100 |
Ta2O5 | Ta | 0.7 | 45 : 5 | 200 | ~200 |
LiAlOxb | Li-Al | 2.0 | 40 : 10 | 200 | ~100 |
WOx | W | 1.0 | 47 : 3 | 70 | 400 |
NiO | Ni | 1.0 | 40 : 10 | 100 | 150 |
ITO | ITO | 0.3 | 100 : 0 | 140 | 400 |
Table 1.
Sputtering parameters for each layer of ECDs
1.2 Characterizations
The section microstructure of ECDs was shown in the cross-section micrographs by field-emission scanning electron microscope (FESEM, FEI Magellan 400). To monitor the surface roughness of monolayer electrolyte film, atomic force microscope (AFM, SII Nano Technology Ltd, Nanonavi P) was examined. Later, the electrochromic property indexes including coloration efficiency, optical contrast, and cycle durability were studied by a CS350 electrochemical workstation (Corrtest, Wuhan). Lastly, UV/Vis/NIR Spectrometer (Hitachi, UV-4100) was used to characterize the in-situ transmittance spectrum of ECDs ranging from 350 to 2600 nm with the scanning speed of 1200 nm/min. In addition, optical modeling was executed via software of Essential Macleod to optimize the experimental spectra, based on the theory of optical interference by utilizing the optical constants of LiAlOx and Ta2O5.
2 Results and discussion
2.1 Designed microstructure of ECDs
The surface microstructure of as-prepared electrolyte was characterized by AFM. The as-deposited LiAlOx and Ta2O5 films exhibited smooth surface with root-mean- square roughness (RMS) of 0.45 and 1.14 nm, respectively (Fig. 1(a-b)). The ECD containing LiAlOx/ Ta2O5/LiAlOx sandwiched electrolyte was fabricated by optical optimization design. The film thickness, local enlarged view and corresponding element distribution of each layer in ECDs can be observed in Fig. 1(c, d, f). In Fig. 1(f), the element distribution diagram corresponds to intuitive expression for the cross-section EDS of ECDs, which represented the element distribution of each layer collated with the SEM photographs in Fig. 1(c). The three middle layers consist of LiAlOx/Ta2O5/LiAlOx sandwich structure with the thickness of nearly 100, 200 and 100 nm, respectively. Besides, surface SEM image of ATA electrolyte display the view of isolated island particles (Fig. 1(e), which indicates the loose porous structure of LiAlOx layer. Thus, the ATA electrolyte constructed the effective conductive channel, which made the most of its excellent properties of ion conduction and stability.
Figure 1.AFM image of LiAlO
2.2 Optical characterization and simulation of ECDs
To study O2 flow ratio dependent optical transmittance of Ta2O5, different O2 contents (O2/(Ar+O2)) (0, 5%, 10% and 15%) were set for Ta2O5 sputtering in Fig. 2(a). When the O2 ratio corresponded to 10%, its optical transmittance was best optimized, which was applied to the sputtering of ATA electrolyte. Fig. 2(b) shows the in-situ transmittance spectra during 350-2600 nm with the applied voltage varying from -3 V to 3 V for ECDs. The initial state was highly bleached. With the increase of positive voltage, the optical transmittance decreased gradually due to its EC coloration effect. Until the bias reaches 3 V, the optical transmittance decreased even below 10%. Especially, ECDs presented excellent color retention as the external voltage was removed. Finally, ECDs returned to its original bleached state when the reverse voltage was applied.
Figure 2.UV-Vis-NIR spectra (a) of Ta2O5 films deposited under different O2 ratios of 0, 5%, 10%, 15%, respectively, optical modulation (b) of ECDs during -3 V to 3 V, experimental (c) and calculated (d) transmittance spectra of sandwich structured electrolyte, in which S1 and S2 refer to two parallel samples, LAO refers to LiAlO
In addition, optical simulation was performed for LiAlOx/Ta2O5/LiAlOx sandwich structured electrolyte. The optical constants (n and k) of LiAlOx and Ta2O5 were used for calculation, which was made for different thickness combinations of LiAlOx and Ta2O5, and an optimized structure was chosen upon the maximum integrated luminous transmittance. Then the experimental and calculated transmittance spectra of sandwich structured electrolyte were tested (Fig. 2(c-d)), which fit well with each other. By means of the optical calculation via software of Essential Macleod, the optical transmittance of ATA reach over 90% at the thickness of 100 nm/ 200 nm/100 nm, which is superior to that of LiTaO3 at the same thickness. The structure of ATA electrolyte was optimized with the optical constants of LiAlOx and Ta2O5 via software of Essential Macleod in Fig. 2(e), which shows the relationship between the luminous transmittance of ATA electrolyte and the film thickness of top and bottom LiAlOx layer, that is, “LiAlOx (bottom layer, thickness t1)/Ta2O5 (200 nm)/ LiAlOx (top layer, thickness t2)”. When t1=t2=0, namely only Ta2O5 monolayer, the visible light transmittance corresponds to the lowest trough. When the top and bottom layer of LiAlOx was introduced, the visible light transmittance increases in a wavy manner and reaches the maximum value over 90% under certain conditions (t1=t2=100 nm). Additionally, the designed structure was calculated according to the transparent state of Ta2O5 when lithium ions locate on the side of electrochromic layer under electric field. Therefore, LiTaO3 (LTO) is equivalent to Ta2O5 (TO), which has little difference in optical constants without lithium ions.
2.3 Comparison of sputtering efficiency for several typical functional layers
One of the highlights of this work is to summarize the sputtering efficiency of electrochromic devices, which is rarely summarized in previous work. Generally speaking, DC power sputtering is relatively more efficient than RF power in the industrial production[
Figure 3.Comparison of sputtering efficiency parameters for several typical layers of ECDs
Layer | Target | Power supply | Power density/(W·cm-2) | Sputtering rate/ | Sputtering efficiency/ | Ref. |
---|---|---|---|---|---|---|
Ta2O5 | Ta2O5 | RF | 0.57 | 2.7 | 4.74 | [23] |
Ta | DC | 0.89 | 15 | 16.85 | This work | |
LiTaO3 | LiTaO3 | RF | 0.48 | 1.2 | 2.50 | [24] |
LiAlOx | Li-Al | DC | 0.16 | 1 | 6.25 | This work |
WOx | WO3 | RF | 1.78 | 5 | 2.81 | [25] |
W | DC | 0.40 | 16 | 40 | This work | |
NiO | NiO | RF | 0.64 | 3.6 | 5.63 | [26] |
Ni | DC | 0.32 | 5 | 15.63 | This work |
Table 2.
Comparison of sputtering efficiency for several typical systems
2.4 Electrochemical property of the ATA based ECDs
As shown in Fig. 4(a), by means of optical design, the optical transmittance of the sandwich structured electrolyte (ATA) has been greatly improved on the premise that the ionic conductivity is not lower than that of LiTaO3 at the same thickness. The designed ATA based ECDs have satisfactory coloring efficiency of 79.6 cm2/C while changing color reversibly (Fig. 4(b)). The inset of Fig. 4(b) reflects reversible color change between the bleached and colored states of the as-prepared ECD with the size of 20 mm×20 mm. Moreover, its response time for coloring and bleaching is as short as 1.9 and 1.6 s, respectively (Fig. 4(c)), which is mainly due to the convenient transport channels and rich ion reservation of ATA sandwich structured electrolyte. At the meantime, it keeps stable optical modulation after 200 cycles with in-situ testing units fixed wavelength at 630 nm (Fig. 4(d)).
Figure 4.Schematic diagram of the ATA based seven-layer all-solid-state ECDs by optical design (a), color efficiency of ECDs with inset correspond to EC reaction of ECDs (b), switching speed (c) and cycle durability test (d) of ECDs
3 Conclusions
In summary, we have developed the sandwich structured electrolyte with high transparency of luminous transmittance over 90% and remarkable sputtering efficiency by DC reactive magnetron sputtering using alloy and metal target. Additionally, ATA sandwich structured electrolyte as the ion conducting layer has been introduced into a seven-layer all-solid-state ECD consisting of ITO/NiO/LiAlOx/Ta2O5/LiAlOx/WO3/ITO. Furthermore, ATA-based ECDs realized satisfactory coloration efficiency of 79.6 cm2/C, fast switching speed as short as 1.9 s for coloring and 1.6 s for bleaching, and excellent cycling stability over hundreds of cycles. Hence, ATA-based ECDs by optical design are expected to realize mass production and practical application in the near future.
References
[1] A L S EH, A W M TAN, X CHENG et al. Recent advances in flexible electrochromic devices: prerequisites, challenges, and prospects. Energy Technology, 6, 33-45(2018).
[2] H X JIA, X CAO, P S JIN. Advances in inorganic all-solid-state electrochromic materials and devices. Journal of Inorganic Materials, 35, 511-524(2020).
[3] K THUMMAVICHAI, Y D XIA, Y Q ZHU. Recent progress in chromogenic research of tungsten oxides towards energy-related applications. Progress in Materials Science, 88, 281-324(2017).
[4] Y F YUE, H Z LI, K R LI et al. Preparation and properties of NiO/Pb hybrid electrochromic film. Journal of Inorganic Materials, 32, 949-954(2017).
[6] S L ZHANG, S CAO, T R ZHANG et al. Al3+ intercalation/ deintercalation-enabled dual-band electrochromic smart windows with a high optical modulation, quick response and long cycle life. Energy & Environmental Science, 11, 2884-2892(2018).
[9] M C WANG, M H HSIEH, Y C CHEN et al. All-solid-state electrochromic device integrated with near-IR blocking layer for image sensor and energy-saving glass application. Applied Physics Letters, 109, 123501(2016).
[10] L L XIE, S W ZHAO, Y ZHU et al. High performance and excellent stability of all-solid-state electrochromic devices based on a Li1.85AlO
[11] Y ZHU, L L XIE, T C CHANG et al. High performance all-solid-state electrochromic device based on Li
[12] D ZHOU, D XIE, X H XIA et al. All-solid-state electrochromic devices based on WO3||NiO films: material developments and future applications. Science China-Chemistry, 60, 3-12(2017).
[13] X ZHANG, Y ZHAO, Q LING et al. Study on preparation of LSTP thin film electrolyte by RF magnetron sputtering and electrical properties. Journal of Materials Science: Materials in Electronics, 31, 542-547(2020).
[14] D L XIAO, J TONG, Y FENG et al. Improved performance of all-solid-state lithium batteries using LiPON electrolyte prepared with Li-rich sputtering target. Solid State Ionics, 324, 202-206(2018).
[15] K TAJIMA, Y YAMADA, S BAO et al. Solid electrolyte of tantalum oxide thin film deposited by reactive DC and RF magnetron sputtering for all-solid-state switchable mirror glass. Solar Energy Materials and Solar Cells, 92, 120-125(2008).
[16] E CUCE, S B J R RIFFAT, S E REVIEWS. A state-of-the-art review on innovative glazing technologies. Renewable Sustainable Energy Reviews, 41, 695-714(2015).
[17] R BAETENS, B P JELLE, A GUSTAVSEN. Properties, requirements and possibilities of smart windows for dynamic daylight and solar energy control in buildings: a state-of-the-art review. Solar Energy Materials and Solar Cells, 94, 87-105(2010).
[18] Y ZHAO, R XU, X ZHANG et al. Simulation of smart windows in the ZnO/VO2/ZnS sandwiched structure with improved thermochromic properties. Energy Buildings, 66, 545-552(2013).
[19] G YU, X XIE, L PAN et al. Hybrid nanostructured materials for high-performance electrochemical capacitors. Nano Energy, 2, 213-234(2013).
[20] C G GRANQVIST, P LANSÅKER, N MLYUKA et al. Progress in chromogenics: new results for electrochromic and thermochromic materials and devices. Solar Energy Materials and Solar Cells, 93, 2032-2039(2009).
[21] P JIN, G XU, M TAZAWA et al. Design, formation and characterization of a novel multifunctional window with VO2 and TiO2 coatings. Applied Physics A, 77, 455-459(2003).
[22] K TAJIMA, H HOTTA, Y YAMADA et al. Fabrication of solid electrolyte Ta2O5 thin film by reactive DC magnetron sputtering suitable for electrochromic all-solid-state switchable mirror glass. Journal of the Ceramic Society of Japan, 119, 76-80(2011).
[23] C CORBELLA. Influence of the porosity of RF sputtered Ta2O5 thin films on their optical properties for electrochromic applications. Solid State Ionics, 165, 15-22(2003).
[24] B SUN, J WANG, J GOU et al. Influence of thermal annealing on structural and optical properties of RF-sputtered LiTaO3 thin films. Materials Research Express, 6, 026405(2018).
[25] S S KALAGI, S S MALI, D S DALAVI et al. Transmission attenuation and chromic contrast characterization of R.F. sputtered WO3 thin films for electrochromic device applications. Electrochimica Acta, 85, 501-508(2012).
[26] K S AHN, Y C NAH, J H YUM et al. The effect of Ar/O2 ratio on electrochromic response time of Ni oxides grown using an RF sputtering system. Japanese Journal of Applied Physics, 41, L212-L215(2002).
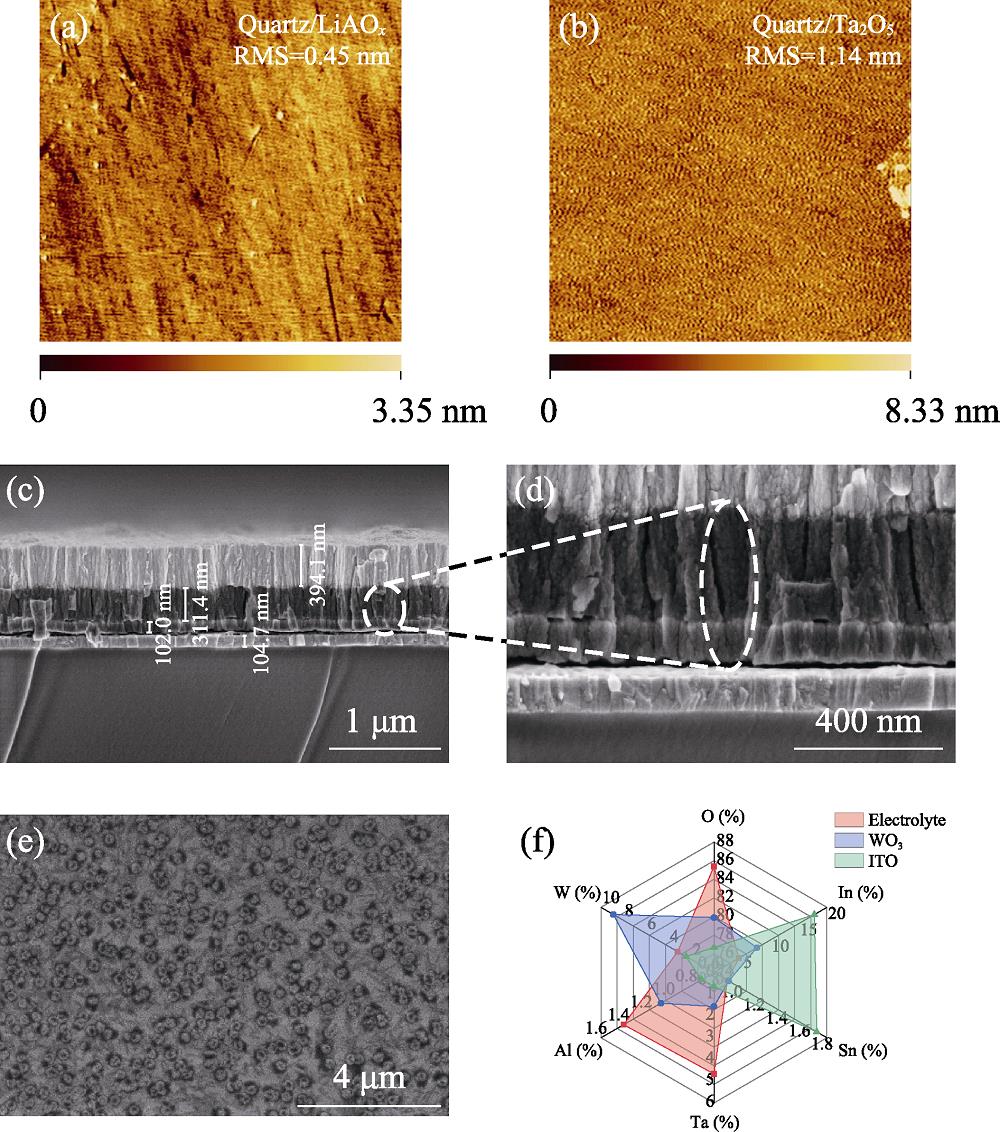
Set citation alerts for the article
Please enter your email address