Author Affiliations
1Huazhong University of Science and Technology, School of Optical and Electronic Information, Wuhan, China2Huazhong University of Science and Technology, Britton Chance Center for Biomedical Photonics, Wuhan National Laboratory for Optoelectronics, Wuhan, China3University of California, Los Angeles, Department of Bioengineering, Los Angeles, California, United States4University of Texas at Arlington, Joint Department of Bioengineering of UT Arlington/UT Southwestern, Arlington, Texas, United States5University of California, Los Angeles, School of Medicine, Los Angeles, California, United States6University of California, Los Angeles, Chemical and Biomolecular Engineering Department, Los Angeles, California, United States7Osaka Prefecture University, Nanoscience and Nanotechnology Research Center, Research Organization for the 21st Century, Osaka, Japan8University of California, Los Angeles, Mechanical and Aerospace Engineering Department, Los Angeles, California, United Statesshow less
![Principles of SLSM. (a) SLSM geometry with wide laser-sheet illumination and large-FOV detection. The sample is scanned in one direction (red arrow) with the deviation angle θ being 10 deg to 20 deg relative to the z axis. (b) Sequence of spatially modulated, LR images recorded under high-frame rate. The step size s is much smaller than the laser-sheet longitudinal extent l(1/e2). The raw image stack is split into multiple substacks (indicated by different colors), with a voxel depth d that is one-third the laser-sheet thickness l. Each LR substack Pk is correlated to the first reference stack P1 (red), with a nonaxial, subvoxel shift sk=(k−1)*s. For instance, P2 (yellow) can be registered to P1 with unit displacement s, which consists of 3-D components sx, sy, and sz simultaneously. (c) Block diagram of iterative SVR procedure. The spatially correlated, wide-view, LR stacks (step 1) are input to an MLE (step 2) to iteratively reconstruct a voxel-super-resolved image. Block Gk represents a gradient backprojection operator that compares the k’th LR image to the estimate of the HR image in the m’th steepest descent iteration. At the final step (3), voxel realignment is applied to recover the sample shape accurately from the slight deformation caused by the tilted scan. (d) Imaging of 500-nm fluorescent beads using SLSM (4×/0.13-DO/0.022-NA sheet illumination), SPIM (10×/0.3-DO/0.04-NA sheet illumination, 20×/0.45-DO/0.06-NA sheet illumination), and confocal microscope (Zeiss LSM 510, 20×/0.7 objective). The x to z planes of the bead are shown to compare the lateral and axial resolving powers of the different methods. (e) and (f) Intensity plots of linecuts [as shown in (d)] through lateral and axial extents of bead for each method, with 50% intensity level (dashed line) shown for the estimation of the FWHM.](/richHtml/ap/2019/1/1/016002/img_001.png)
Fig. 1. Principles of SLSM. (a) SLSM geometry with wide laser-sheet illumination and large-FOV detection. The sample is scanned in one direction (red arrow) with the deviation angle being 10 deg to 20 deg relative to the axis. (b) Sequence of spatially modulated, LR images recorded under high-frame rate. The step size is much smaller than the laser-sheet longitudinal extent . The raw image stack is split into multiple substacks (indicated by different colors), with a voxel depth that is one-third the laser-sheet thickness . Each LR substack is correlated to the first reference stack (red), with a nonaxial, subvoxel shift . For instance, (yellow) can be registered to with unit displacement , which consists of 3-D components , , and simultaneously. (c) Block diagram of iterative SVR procedure. The spatially correlated, wide-view, LR stacks (step 1) are input to an MLE (step 2) to iteratively reconstruct a voxel-super-resolved image. Block represents a gradient backprojection operator that compares the ’th LR image to the estimate of the HR image in the ’th steepest descent iteration. At the final step (3), voxel realignment is applied to recover the sample shape accurately from the slight deformation caused by the tilted scan. (d) Imaging of 500-nm fluorescent beads using SLSM (4×/0.13-DO/0.022-NA sheet illumination), SPIM (10×/0.3-DO/0.04-NA sheet illumination, 20×/0.45-DO/0.06-NA sheet illumination), and confocal microscope (Zeiss LSM 510, 20×/0.7 objective). The to planes of the bead are shown to compare the lateral and axial resolving powers of the different methods. (e) and (f) Intensity plots of linecuts [as shown in (d)] through lateral and axial extents of bead for each method, with 50% intensity level (dashed line) shown for the estimation of the FWHM.
![SLSM demonstration of 3-D cultured NHBE cells. (a) NHBE cell spheroids 3-D cultured in a piece of Matrigel substrate. The cells were fluorescently labeled through DAPI nuclear staining for SLSM reconstruction. (b1) LR raw image of selected cell spheroid (first of 64 groups), captured under illumination and detection NAs of 0.022 and 0.13, respectively, with 1.625-μm×1.625-μm×6-μm voxel size. The vignette views show a single-cell nucleus. (b2) Initial estimate of SVR computation, which is a 4× interpolation of b1. (b3) Final SVR reconstruction of the same region, with a reconstructed voxel size of 0.41 μm×0.41 μm×1.5 μm. The features of the cell nucleus were recovered through blurring and pixelation, with the chromatin becoming discernible. (c) Images of same cell spheroid taken by SPIM using 20×/0.45 objective and 0.06-NA illumination, for comparison. (d) Intensity plot of linecuts [shown in (b) and (c)] for each setting, indicating that SVR provides substantively improved contrast and resolution. (e) Volume renderings in raw mode and SVR reconstruction, comparing 3-D localization accuracy of single cells in self-assembled spheroid. Scale bars: 20 μm (5 μm in insets). (f) Comparison of imaging resolution, speed, and photobleaching for 4×-SPIM, 10×-SPIM tile imaging, 20×-SPIM tile imaging, 20×-confocal microscopy, and 4×-SLSM. The achieved effective throughputs are 2.7, 1.7, 0.9, 0.07, and 24 megavoxel SBP per second, respectively.](/richHtml/ap/2019/1/1/016002/img_002.png)
Fig. 2. SLSM demonstration of 3-D cultured NHBE cells. (a) NHBE cell spheroids 3-D cultured in a piece of Matrigel substrate. The cells were fluorescently labeled through DAPI nuclear staining for SLSM reconstruction. (b1) LR raw image of selected cell spheroid (first of 64 groups), captured under illumination and detection NAs of 0.022 and 0.13, respectively, with voxel size. The vignette views show a single-cell nucleus. (b2) Initial estimate of SVR computation, which is a interpolation of b1. (b3) Final SVR reconstruction of the same region, with a reconstructed voxel size of . The features of the cell nucleus were recovered through blurring and pixelation, with the chromatin becoming discernible. (c) Images of same cell spheroid taken by SPIM using 20×/0.45 objective and 0.06-NA illumination, for comparison. (d) Intensity plot of linecuts [shown in (b) and (c)] for each setting, indicating that SVR provides substantively improved contrast and resolution. (e) Volume renderings in raw mode and SVR reconstruction, comparing 3-D localization accuracy of single cells in self-assembled spheroid. Scale bars: ( in insets). (f) Comparison of imaging resolution, speed, and photobleaching for 4×-SPIM, 10×-SPIM tile imaging, 20×-SPIM tile imaging, 20×-confocal microscopy, and 4×-SLSM. The achieved effective throughputs are 2.7, 1.7, 0.9, 0.07, and 24 megavoxel SBP per second, respectively.
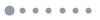
Fig. 3. Rapid high-throughput volumetric imaging of whole organisms via SLSM. (a) 91-gigavoxel SVR reconstruction of optically cleared intact mouse heart (inset: neonate day 1) with endogenous autofluorescence. The magnified views of the slices along the indicated coronal and axial planes (i) and (ii) reveal that the SVR procedure substantively improves visualization of the cardiomyocytes compared with the raw images. The image acquisition and SVR reconstruction time for the entire heart were and 12 min, respectively. Scale bars: 1 mm (magnified views: ). (b) Two-color, 34-gigavoxel SVR reconstruction of anesthetized live zebrafish embryo (3 dpf) tagged with Islet1-GFP at motor neurons and mlcr-DsRed at somite fast muscle; (b2) magnified volume rendering in SVR reconstruction of fish somite section, elucidating the interplay of the motor neurons with the muscles during development. The right-side images show slices through the somite along the planes shown in the image on the left. The SLSM image acquisition was completed in 120 s for dual colors, followed by 200 s of GPU-based processing. Scale bars: for the whole embryo image (magnified views: ).
![mv-SLSM for 3-D isotropic imaging of large scattering HUVEC-HDF cell sprouting network. (a) The mv-SVR procedure involves the following steps: (1) image acquisition under multiple views, (2) SVR computation for each view, (3) image registration in 3-D space, and (4) weighted mv-SVR fusion with final deconvolution. (b) 3-D reconstruction of cells obtained using a single-view SPIM (2×/0.06-DO/0.015-NA sheet-illumination) and four-view SLSM using the same optics. The SPIM system SBP is 1.1 gigavoxel for the entire sample, whereas four-view SLSM creates a 190-gigavoxel SBP that renders a higher resolution and more complete sample structure across a large FOV of ∼100 mm3. The vignette HR views compare visualizations of the HUVEC branch, tip filopodia, and HDF colocalization (white arrowheads). (c) and (d) Maximum intensity projections of supporting HDF cells in x to y and x to z planes. mv-SVR reconstruction enables clear identification of single fiber sprouting [right columns of (c) and (d)], which remains very fuzzy in the single-view SPIM image [left columns of (c) and (d)]. The intensity plot of the blue linecut [inset in (d)] indicates a small resolvable distance of ∼1.6 μm. In addition to the isotropic resolution enhancement, mv-SVR reconstruction also restores the highly scattered area that is originally subject to severe signal loss in the single-view image [left area in (d)]. Scale bars: 1 mm in (a) and (b) (magnified views: 100 μm) and 100 μm in (c) and (d) (magnified vignette views: 10 μm).](/Images/icon/loading.gif)
Fig. 4. mv-SLSM for 3-D isotropic imaging of large scattering HUVEC-HDF cell sprouting network. (a) The mv-SVR procedure involves the following steps: (1) image acquisition under multiple views, (2) SVR computation for each view, (3) image registration in 3-D space, and (4) weighted mv-SVR fusion with final deconvolution. (b) 3-D reconstruction of cells obtained using a single-view SPIM (2×/0.06-DO/0.015-NA sheet-illumination) and four-view SLSM using the same optics. The SPIM system SBP is 1.1 gigavoxel for the entire sample, whereas four-view SLSM creates a 190-gigavoxel SBP that renders a higher resolution and more complete sample structure across a large FOV of . The vignette HR views compare visualizations of the HUVEC branch, tip filopodia, and HDF colocalization (white arrowheads). (c) and (d) Maximum intensity projections of supporting HDF cells in to and to planes. mv-SVR reconstruction enables clear identification of single fiber sprouting [right columns of (c) and (d)], which remains very fuzzy in the single-view SPIM image [left columns of (c) and (d)]. The intensity plot of the blue linecut [inset in (d)] indicates a small resolvable distance of . In addition to the isotropic resolution enhancement, mv-SVR reconstruction also restores the highly scattered area that is originally subject to severe signal loss in the single-view image [left area in (d)]. Scale bars: 1 mm in (a) and (b) (magnified views: ) and in (c) and (d) (magnified vignette views: ).
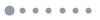
Fig. 5. Three-dimensional neuronal mapping of adult mouse brain via an eight-view SLSM. (a) Isotropic SVR volume rendering of optically cleared Thy1-GFP-M mouse brain block (350-gigavoxel scale; scale bar: ). (b), (c), and (d1) HR volumetric views of hippocampus, thalamus, and cortex regions, respectively. (d2) Cortex reconstructed using regular SPIM with 4×/0.13-DO plus 0.022-NA PI, for comparison (scale bar: ). (e) Analysis of neural structures and connectivity. Three clustered astrocyte neurons in the thalamus region are segmented with different colors encoded in (i). Three registered projection neurons across the brain block are annotated according to the mice brain atlas (ii, iii, and iv). Abbreviations: cg, cingulum; ec, external capsule; dhc, dorsal hippocampal commissure; CPu, caudate putamen; ic, internal capsule; Rt, reticular thalamic nucleus; Prs, presubiculum; RSG, retrosplenial granular cortex; S1HL, primary somatosensory cortex hindlimb; RSA, retrosplenial agranular cortex; and scale bar: .