
- Chinese Optics Letters
- Vol. 20, Issue 1, 012201 (2022)
Abstract
Keywords
1. Introduction
UV-inscribed fiber Bragg gratings (FBGs) are ideal optical devices in telecom, lasers, and sensing systems. The phase mask scanning technique offers high quality FBG fabrication with high reproducibility and ease of design. So far, most of the FBGs reported using a phase mask scanning method are based on the first order Bragg diffraction of the fiber grating structure. It is well known that due to the saturation effect of the UV irradiation, high order Bragg resonance can be observed in a non-tilted uniform FBG[
2. Theoretical and Experimental Analysis
The Bragg diffraction of a uniform FBG is defined as
According to the Kramers–Kronig relationship[
Sign up for Chinese Optics Letters TOC. Get the latest issue of Chinese Optics Letters delivered right to you!Sign up now
Figure 1(a) shows the simulation result of the normalized index change curve at different UV-exposure time. When the index change gets saturated, the refractive index profile tends to be a square shape, which results in high order Fourier components. The refractive index change can be expanded as a Fourier series, which expressed as
Figure 1.(a) Simulation of normalized index change at different exposure time; (b) simulation of second order reflectivity against exposure time.
According to the coupled mode theory, the maximum reflectivity of the
A set of TFGs were UV-inscribed in B/Ge co-doped commercial photosensitive fiber (Fibercore PS1200/1500) using the scanning phase mask technique and a 244 nm UV source from a CW frequency doubled
In Fig. 2(a), the plot depicts the measured 2nd-OBR wavelength of each TFG against the tilt angle. The results show very good accordance with the theoretical simulation. As the angle increases, the resonance wavelength has a corresponding red shift. We also analyzed the grating strength against the tilt angle, which is shown in Fig. 2(b). Under the same UV illumination power and exposure time, it is found that the larger the tilt angle is, the stronger the 2nd-OBR is. At certain angles, especially small angles, multiple scanning may have to be employed to make the 2nd-OBR effect more pronounced. This is because when the grating is slightly tilted, the grating refractive index profile tends to be more sinusoidal, and hence the higher order resonance will not be strong enough to observe.
Figure 2.(a) Experimental and theoretical relationship between the 2nd-OBR wavelength and the grating tilt angle; (b) 2nd-OBR strength against the tilt angle.
Previously, it was reported that when the grating tilted angle is 45° during the UV-inscription procedure, the TFG exhibits stronger polarization dependent loss (PDL) over a wide range than other tilted angles[
Figure 3.Measured PDL spectra of the corresponding grating within 30 nm spectrum range showing 2nd-OBR in both p polarization and
3. Application of Single Polarization Fiber Laser
Erbium-doped fiber lasers are useful light sources in telecom and sensing systems where single polarization and single wavelength operation is desirable. There are numerous ways to produce polarized output laser. However, they use either expensive specialty fibers[
The fiber laser construction is shown in Fig. 4, which consists of an
Figure 4.Schematic of the demonstrated fiber laser using the 2nd-OBR generated by a 45° TFG.
The experiment results show that the laser has output a single wavelength laser at
Figure 5.(a) Typical output spectrum of the fiber laser; (b) slope efficiency of the fiber laser.
Figure 6.(a) PER spectra of the laser; (b) tuning ability demonstration of the fiber laser; (c) temperature sensitivity of the fiber laser; (d) output wavelength and amplitude variation of the fiber laser within 1 h in laboratory conditions.
4. Conclusions
In conclusion, we have theoretically and experimentally investigated the 2nd-OBRs of the TFG. Simulation results revealed that the square-shape index modulation profile could enhance the second Bragg resonance of TFG. In the experiment, we have observed the 2nd-OBR of TFG with the tilt angles of 0°, 12.3°, 22°, 30.6°, 36.9°, and 45°. The wavelength of 2nd-OBR of 45° TFG is located just inside of the polarizing bandwidth of 45° TFG, which induces very strong polarization dependent resonance. The 2nd-OBR of 45° TFG would be an ideal polarizing FBG. Based on this, we have achieved a polarized fiber laser system, in which the laser output shows high DOP (
References
[1] W. X. Xie, M. Douay, P. Bernage, P. Niay, J. F. Bayon, T. Georges. Second order diffraction efficiency of Bragg gratings written within germanosilicate fibres. Opt. Commun., 101, 85(1993).
[2] G. P. Brady, K. Kalli, D. J. Webb, D. Jackson, L. Reekie, J. L. Archambault. Simultaneous measurement of strain and temperature using the first and second-order diffraction wavelengths of Bragg gratings. IEE Proc. Optoelectron., 144, 156(1997).
[3] J. Echevarria, A. Quintela, C. Jauregui, J. M. López-Higuera. Uniform fiber Bragg grating first- and second-order diffraction wavelength experimental characterization for strain-temperature discrimination. IEEE Photon. Technol. Lett., 13, 696(2001).
[4] S. Li, D. Hua, L. Hu, Q. Yan, X. Tian. All-fiber spectroscope with second-order fiber Bragg grating for rotational Raman lidar. Spectrosc. Lett., 47, 244(2013).
[5] J. Albert, L.-Y. Shao, C. Caucheteur. Tilted fiber Bragg grating sensors. Laser Photon. Rev., 7, 83(2013).
[6] X. Guo, Z. Xing, H. Qin, Q. Sun, D. Liu, L. Zhang, Z. Yan. Study on polarization spectrum and annealing properties of 45°-tilted fiber gratings. Chin. Opt. Lett., 17, 050601(2019).
[7] Z. Yan, C. Mou, K. Zhou, X. Chen, L. Zhang. UV-inscription, polarization-dependant loss characteristics and applications of 45° tilted fiber gratings. J. Lightwave Technol., 29, 2715(2011).
[8] Y. Sun, Z. Yan, K. Zhou, B. Luo, B. Jiang, C. Mou, Q. Sun, L. Zhang. Excessively tilted fiber grating sensors. J. Lightwave Technol., 39, 3761(2021).
[9] B. Luo, H. Lu, S. Shi, M. Zhao, J. Lu, Y. Wang, X. Wang. Plasmonic gold nanoshell induced spectral effects and refractive index sensing properties of excessively tilted fiber grating. Chin. Opt. Lett., 16, 100603(2018).
[10] Y. Moreno, Q. Song, Z. Xing, Y. Sun, Z. Yan. Hybrid tilted fiber gratings-based surface plasmon resonance sensor and its application for hemoglobin detection. Chin. Opt. Lett., 18, 100601(2020).
[11] T. Lu, Y. Sun, Y. Moreno, Q. Sun, K. Zhou, H. Wang, Z. Yan, D. Liu, L. Zhang. Excessively tilted fiber grating-based vector magnetometer. Opt. Lett., 44, 2494(2019).
[12] Z. Huang, Q. Huang, A. Theodosiou, X. Cheng, C. Zou, L. Dai, K. Kalli, C. Mou. All-fiber passively mode-locked ultrafast laser based on a femtosecond-laser-inscribed in-fiber Brewster device. Opt. Lett., 44, 5177(2019).
[13] T. Wang, Z. Yan, C. Mou, Z. Liu, Y. Liu, K. Zhou, L. Zhang. Narrow bandwidth passively mode locked picosecond erbium doped fiber laser using a 45° tilted fiber grating device. Opt. Express, 25, 16708(2017).
[14] B. Lu, C. Zou, Q. Huang, Z. Yan, Z. Xing, M. Al Araimi, A. Rozhin, K. Zhou, L. Zhang, C. Mou. Widely wavelength-tunable mode-locked fiber laser based on a 45°-tilted fiber grating and polarization maintaining fiber. J. Lightwave Technol., 37, 3571(2019).
[15] X.-Y. Zhang, H.-B. Sun, C. Chen, Y.-S. Yu, W.-H. Wei, Q. Guo, Y.-Y. Chen, X. Zhang, L. Qin, Y.-Q. Ning. High-order-tilted fiber Bragg gratings with superposed refractive index modulation. IEEE Photon. J., 10, 7100308(2018).
[16] C. Mou, R. Suo, K. Zhou, L. Zhang, I. Bennion. 2nd order Bragg resonance generated in a 45° tilted fiber grating and its application in a fiber laser. Advanced Photonics & Renewable Energy, OSA Technical Digest (CD), BTuA7(2010).
[17] T. Erdogan. Fiber grating spectra. J. Lightwave Technol., 15, 1277(1997).
[18] Y. D. Gong, T. J. Li, Q. Cai, Q. Li, Z. Wang, Y. L. Guan, J. S. Zhang, S. S. Jian. Novel B/Ge codoped photosensitive fiber and dispersion compensation in an 8×10 Gbit/s DWDM system. Opt. Laser Technol., 32, 23(2000).
[19] M. Kashiwagi, K. Takenaga, K. Ichii, T. Kitabayashi, S. Tanigawa, K. Shima, S. Matsuo, M. Fujimaki, K. Himeno. Over 10 W output linearly-polarized single-stage fiber laser oscillating above 1160 nm using Yb-doped polarization-maintaining solid photonic bandgap fiber. IEEE J. Quantum Electron., 47, 1136(2011).
[20] J. Xu, S. Wu, J. Liu, Y. Li, J. Ren, Q. Yang, P. Wang. All-polarization-maintaining femtosecond fiber lasers using graphene oxide saturable absorber. IEEE Photon. Technol. Lett., 26, 346(2014).
[21] M. S. Astapovich, A. V. Gladyshev, M. M. Khudyakov, A. F. Kosolapov, M. E. Likhachev, I. A. Bufetov. Watt-level nanosecond 4.42-µm Raman laser based on silica fiber. IEEE Photon. Technol. Lett., 31, 78(2019).
[22] D. Pureur, M. Douay, P. Bernage, P. Niay, J. F. Bayon. Single-polarization fiber lasers using Bragg gratings in Hi-Bi fibers. J. Lightwave Technol., 13, 350(1995).
[23] S. Liu, F. Yan, W. Peng, T. Feng, Z. Dong, G. Chang. Tunable dual-wavelength thulium-doped fiber laser by employing a HB-FBG. IEEE Photon. Technol. Lett., 26, 1809(2014).
[24] C. Mou, K. Zhou, L. Zhang, I. Bennion. Characterization of 45°-tilted fiber grating and its polarization function in fiber ring laser. J. Opt. Soc. Am. B, 26, 1905(2009).
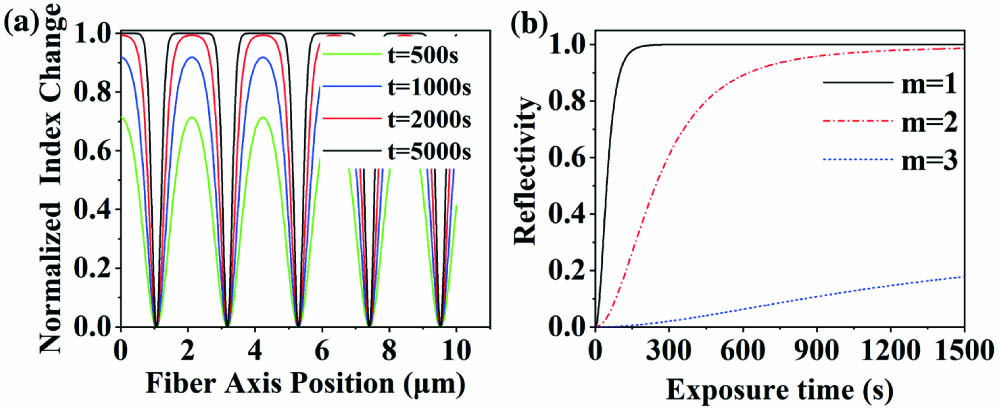
Set citation alerts for the article
Please enter your email address