Author Affiliations
1Xi’an Institute of Optics and Precision Mechanics of Chinese Academy of Sciences, Center for Attosecond Science and Technology, State Key Laboratory of Transient Optics and Photonics, Xi’an, China2University of Chinese Academy of Sciences, Beijing, China3Shanxi University, Collaborative Innovation Center of Extreme Optics, Taiyuan, Chinashow less
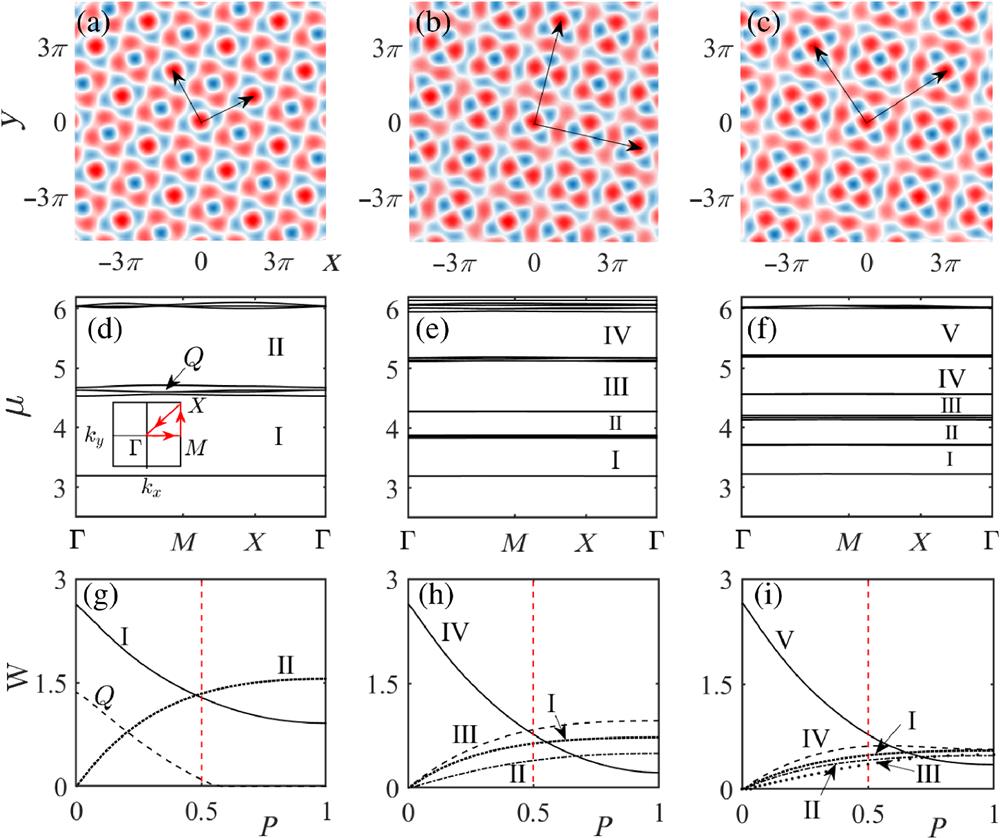
Fig. 1. Linear Bloch-wave band structures of 2D moiré square optical lattices. The geometries, reduced Brillouin zone, and bandgap spectra of the 2D moiré square optical lattices under different Pythagorean twisted angles. Platforms of the moiré lattice (shaded red, lattice potential maxima; shaded blue, lattice potential minima) at different rotation angles: (a) , (b) , and (c) , and the linear Bloch-wave spectrum (indicated as chemical potential ) of the moiré lattice with different twisted angles: (d) , (e) , and (f) ; subplot in panel (d) shows the first reduced Brillouin zone in the reciprocal space, where the associated high symmetry points are marked. The width of the finite gaps versus the relative strength of two layers of the lattice () under different twisted angles: (g) , (h) , and (i) . The second and third lines: the Roman numerals I, II, III, IV, and V are the first, second, third, fourth, and fifth finite gaps, respectively; in panels (d) and (g) is the very narrow minigap. for panels (a)–(f).
Fig. 2. Properties of fundamental gap solitons of ultracold Fermi gases controlled by moiré square optical lattices. Number of atoms, , versus chemical potential (a)–(c), respectively, and the same sublattice depth () under (d) for the fundamental gap solitons, whose profiles for marked points (, , and ) in panel (a) are shown in the third line, in 2D moiré square optical lattices at twisted angles: (a), (d) , (b) , and (c) . Other parameters: (d) ; (e) , ; (f) , ; (g) , . Here and below, red dashed lines in panels (a)–(c) represent unstable regions.
Fig. 3. Perturbed evolutions of fundamental gap solitons. Top-view profiles (a)–(c) and the perturbed evolution in real time (d)–(f) of typical fundamental gap solitons for the marked points (, , and ) in Fig. 2(a): stable (a), weakly unstable (b), and strongly unstable (c) gap solitons.
Fig. 4. Properties of gap vortices of ultracold Fermi gases loaded upon moiré square optical lattices. Number of atoms, , versus chemical potential (a)–(c) and the same sublattice depth () under (d) for the vortex gap solitons at topological charge in 2D moiré square optical lattices at twisted angles: (a), (d) , (b) , and (c) . Other parameters: (d) ; (e) , ; (f) , ; (g) , . Red dashed lines in panels (a)–(c) represent unstable regions. The cross section (taken at ), the associated profiles, and phase structures are depicted in the third, fourth, and fifth lines, respectively.
Fig. 5. Perturbed evolutions of gap vortices. Top-view profiles (a)–(c) and the perturbed evolution in real time (d)–(f) of typical vortex gap solitons for the marked points (, , and ) in Fig. 4(a): stable (a), weakly unstable (b), and strongly unstable (c) gap vortices.