Author Affiliations
1State Key Laboratory of Modern Optical Instrumentation, College of Optical Science and Engineering, Zhejiang University, Hangzhou 310027, China2College of Electronics and Information Engineering, Shanghai University of Electric Power, Shanghai 200090, China3Collaborative Innovation Center of Extreme Optics, Shanxi University, Taiyuan 030006, China4Research Center for Intelligent Sensing, Zhejiang Lab, Hangzhou 311100, China5e-mail: liuyongdx@shiep.edu.cn6e-mail: cfkuang@zju.edu.cnshow less
Fig. 1. (a) Lateral and axial PSFs of 3D diffraction-limited dark spot of hollow excitation beam (Ihexc). (b) The lateral and axial PSFs of 3D sub-diffraction dark spot (SDS). (c) Numerical imaging comparison of Iexc,ISTED, and ISDS. The simulative areas are all 2 μm×2 μm with 10-nm pixel. (d) Normalized intensity curves of hollow depletion beam (Idep), ISTED, and ISDS. (e) The MTF analysis of Iexc,Ihexc, ISTED, and ISDS. The spatial frequency values are normalized by 2π NA/λ. NA: 1.4;λ: 532 nm; Iexc: 10 kW/cm2; Ihexc: 10 kW/cm2; Idep: 5 MW/cm2. (f) The working principle comparison between MINFLUX (upper row) and SDLM (lower row). The depletion beam intensities of 50–100 MW/cm2 are applied. wf, wide field. (g) The schematic of the optical setup of SDLM. The four deflections of the excitation pattern in specific positions are called excitation beam patterning (EBP). OL, objective lens. Scale bars in all: 200 nm.
Fig. 2. PSFs of ISDS with different Idep for 3D SDS. (a) The PSFs of ISDS for lateral dark spots in the lateral plane (Idep=1–7 MW/cm2). (b) The dependence of the FWHM and oFWHM on Idep for lateral dark spots (Idep=1–9 MW/cm2). (c) The PSFs of ISDS for axial dark spots in the axial plane (Idep=1–7 MW/cm2). (d) The dependence of the FWHM and oFWHM on Idep for axial dark spots (Idep=1–9 MW/cm2).
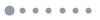
Fig. 3. (a) CRB values in the lateral localization for the origin of the lateral detection circle when Lxy=4–20 nm. (b) The statistics histogram of CRB for the origin of the lateral detection circle when N=50–350. The inset top-right graph shows the beam deflections in four specific positions in the XY plane. (c) CRB color mapping of SDLM via lateral localization for the whole region of the detection circle in the XY plane when Lxy=20 nm, N=50, and SBR=30. (d) The CRB values in the axial localization for the origin of the axial detection when Lz=4–20 nm. (e) The statistics histogram of CRB for the origin of the axial detection when N=50–35. The inset top-right graph shows the beam deflections in three specific positions in the Z-axis direction. (f) CRB curves of SDLM via Z-axis localization along the whole axial detection length when Lz=20 nm, N=50–350, and SBR=30. Idep=30 MW/cm2 is applied for the above-mentioned SDLM. (g), (h) The influence of background noise on the lateral and axial CRB values when N=50.
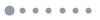
Fig. 4. (a) Lateral CRB comparison (in the origin of the detection circle) between different sizes of SDS: oFWHM of ∼50 nm, ∼100 nm, ∼150 nm, and ∼200 nm, respectively, while different depletion powers are applied when L=10–40 nm and N=50. (b) The axial CRB comparison between different sizes of SDS when L=10–40 nm and N=50. (c) Root mean square error (RMSE) comparison between SDLM methods with different oFWHM values based on the 11 evenly distributed test points on the diagonal of the cylinder in the 3D case. (d) Localization result of SDLM on 3D randomly distributed molecule concentration ambients. The photon number is 200 used in those simulations. Each XY plane contains 30 random molecules. Five XY planes and five XZ planes are selected for observation. It is clearly seen that SDLM can well restore the distribution characteristics of scattered points. (e), (f) RMSE quantitative analysis of molecules either on the lateral plane or on the axial plane.
Fig. 5. (a) Localization results of SDLM on the 3D letter “A” shaped map. The photon number is 200 in those simulations while L=20 nm. (b), (c) RMSE quantitative analysis of molecules either on the lateral plane or on the axial plane.