Fig. 1. Illustration of various GO precursors reduced by laser scrbing. (
a) The preparative procedure of LSG microcircuit on GO film. (
b–c) Optical microscopy images of laser patterned microcircuit; Scale bars, 10 μm. (
d) Atomic force microscope (AFM) image of LSG microcircuit on GO film, the height profile along the white line (L2), and its 3D image. (
e) Survey X-ray photoelectron spectra of GO and LSG. Inset is a photograph of a LSG square on a GO film. (
f) C1s x-ray photoelectron spectroscopy (XPS) spectra of GO and LSG. (
g) The experimental setup of pulsed laser reduction system. The inset is optical images of GO solution (15 mL 0,1 mg/mL) before (i) and after (ii) pulsed laser irradiation. (
h) Schematic illustration of the GO aerogel treated by laser for the preparation of graphene bulks. Figures reproduced with permission from: (a–e) ref.
33 and (g) ref.
43, Elsevier; (h) ref.
45, John Wiley and Sons.
Fig. 2. (
a) Schematic illustration of laser patterning on polyimide (PI). (
b–c) SEM image of LSG on the PI substrate (b) with scale bar 1 mm and its enlarged SEM image with scale bar 10 μm (c). Inset is the corresponding SEM image with higher magnification, scale bar 1 μm. (
d–e) Raman spectrum (d) and X-ray diffraction (XRD) (e) of a LSG and the PI film. (
f) A photo of LSG on pine wood. (
g) The letter “R” in LSG induced from bread. (
h) Picture of LSG patterned into an “R” on a coconut (2 cm tall). (
i–j) LSG on cloth in the shape of an owl (60 mm in height) (i) and the wrapped cloth (j). Figure reproduced with permission from: (a–e) ref.
48, Springer Nature; (f) ref.
52, John Wiley and Sons; (g–j) ref.
53, American Chemical Society.
Fig. 3. (
a) Schematic illustration of the fabrication of LSG based supercapacitors with sandwiched structures. (
b) Schematic diagram of the preparation process for an in-plane LSG supercapacitor. Figure reproduced with permission from: (a) ref.
69 , AAAS; (b) ref.
70 , Springer Nature.
Fig. 4. (
a) Synthetic scheme for the preparation of boron-doped LSG and its fabrication of supercapacitor. (
b) The B1s spectrum of XPS spectra of PI/H
3BO
3 sheet and boron-doped LSG. (
c–d) Cyclic voltammetry curves (c) and galvanostatic charge-discharge curves (d) of LSG SC and boron-doped LSG SC with different H
3BO
3 loadings. Figure reproduced with permission from ref.
72 , American Chemical Society.
Fig. 5. (
a) Schematic showing the insertion of CNTs between GO layers to effectively inhibit the restacking and the fabrication process for the flexible supercapacitor (LSG/CNTs SC). (
b) Digital photographs of an assembled SC. (
d–e) Cyclic voltammetry curves (d), charge-discharge curves (e) and for LSG SCs, LSG/CNTs SCs with different diameters. (
f) Schematic illustration and photos of fabrication of LSG/Au supercapacitors onto a paper substrate. (
g) The SEM image of LSG/Au microelectrodes. (
h–i) Comparison of electrochemical performances of both the LSG/Au SCs and LSG SCs: cyclic voltammetry curves (h) and galvanostatic charge/discharge curves (i). Figure reproduced with permission from: (a–e) ref.
73, Elsevier; (f–i) ref.
71, Royal Society of Chemistry.
Fig. 6. (
a) Schematic diagram of a high-voltage planar SC based on laser scribed graphene. (
b) Schematic illustration and the related strain property of the kirigami-inspired electrodes with different geometric unit numbers (scale bar 1 cm). (
c) Illustration of stacked LSG-SCs in series and parallel circuits and its structure. (
d) Schematic diagram of the direct laser reduction of GO fiber for the bamboo-like series of GO-LSG fiber. (
e) Bio-inspired fractal electrode design of Hilbert fractal structures. (
f) Schematic structure of the Hilbert fractal electrode supercapacitor. Figure reproduced with permission from: (a) ref.
74, American Chemical Society; (b) ref.
96, Springer Nature; (c) ref.
34, American Chemical Society; (d) ref.
98, Royal Society of Chemistry; (e−f) ref.
75, under a Creative Commons Attribution 4.0 International License.
Fig. 7. Schematic and structural illustration of LSG/Ni-CAT MOF. (
a) An in-plane interdigital LSG pattern. (
b) Solvothermal growth of Ni-CAT MOF nanorods. (
c) Structure of LSG/Ni-CAT MOF. (
d) SEM images of LSG and LSG/Ni-CAT MOFs, respectively. (
e) Cyclic voltammetry comparison of bare LSG and LSG/Ni-CAT MOF. (
f) Galvanostatic charge/discharge curves of bare LSG and LSG/Ni-CAT MOF. Figure reproduced with permission from ref.
35, John Wiley and Sons.
Fig. 8. (
a) Schematic illustration of the deposition process of laser-oxidized Fe
3O
4 nanoparticles anchored on porous laser scribed graphene by direct laser writing technique. (
b–c) SEM images of (
b) 3D porous LSG and (
c) 3D LSG/Fe
3O
4 nanoparticle composite. (
d) CV curves of LSG/Fe
3O
4-X at 5 mV·s
−1 (
e) GCD profiles of LSG/Fe
3O
4-X at 1 mA·cm
−2 . Figure reproduced with permission from ref.
77, Elsevier.
Fig. 9. (
a) Schematic of the fabrication steps for the LSG supercapacitor on textile. (
b) CV measurements on LSG supercapacitors without encapsulation under different stretchable conditions for a scan rate of 5 V·s
−1. (
c) Capacitance retention under the maximum stretchable condition of 200% along the uniaxial direction for a scan rate of 5 V·s
−1. Figure reproduced with permission from ref.
112, under a Creative Commons Attribution 4.0 International License.
Fig. 10. (
a) Schematic of experimental setup using a 405 nm laser in SEM. (
b) Fabrication of integrated micro-supercapacitors on a GO film using fs laser processing. (
c–d) LSG electrode arrays maintain high resolution with a spacing of ~2 μm. (
e–f) CV profiles of fs MSC with the interelectrode spacing of (e) 2 μm and (f) 550 μm. (
g) Two-photon-induced 3D graphene micro-supercapacitor using a fs laser. Figure reproduced with permission from: (a) ref.
117 and (b–f) ref.
80, American Chemical Society; (g) ref.
81, under a Creative Commons Attribution 4.0 International License.
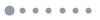
Fig. 11. (
a) Schematic of the integrated energy storage with silicon solar cells. (
b) Schematic illustration of the self-powered photodetection system including a commercial solar panel, a SC, and a ZnO-based photodetector. (
c) Self-discharge curve of the SC after being charged by the solar panel for 1 minute. (
d) Photocurrent curves of the photodetector driven by the SC. (
e) Schematic illustration for the fabrication of a wireless charging and storage integrated device. (
f) Potential change of the integrated SC charged by the wireless circuit placed on a commercial wireless charger. (
g) Serially connected thermally chargeable SC modules whose ends are colored in black and silvery-white to create temperature differences under solar radiation. (
h) The steady-state voltage of 8 thermally chargeable SC modules as a function of Δ
T. Figure reproduced with permission from: (a) ref.
122, AIP Publishing; (b–d) ref.
124, Elsevier; (e, f) ref.
57, American Chemical Society; (g, h) ref.
125, Elsevier.
Laser | Precusor | Substrate | Structure | Electrolyte | Supercapacitor performance | Ref. | Voltage
(V)
| CA(mF·cm−2)
| CV(F·cm−3)
| EV(mWh·cm−3)
| PV(W·cm−3)
| GO: graphene oxide; PI: polyimide; PET: polyethylene terephthalate; PDMS: polydimethylsiloxane; fs: femtosecond; ns: nanosecond; CNTs: carbon nanotubes; PVA: poly(vinyl alcohol); BMIM-BF4: 1-butyl-3-methylimidazolium tetrafluoroborate; TEABF4: tetraethylammonium tetrafluoroborate; FS-IL: fumed silica nanopowder with the IL 1-butyl-3-methylimidazolium bis(trifluoromethyl sulfonyl)imide; BMIM: 1-butyl-3-methyimidazolium bis (trifluoromethyl sulfonyl) imide; Ni-CAT MOF: Ni-catecholate-based metal–organic frameworks; MnO2: manganese dioxide; FeOOH: ferric oxyhydroxide; PANI: polyaniline
| LightScribe DVD
optical drive
| GO film | PET | Sandwich | PVA/H3PO4 | 1 | N/A | 0.4 | 0.04 | 1 | Ref.69 | TEABF4 | 3 | 4.82 | N/A | 0.4 | 10 | BMIM-BF4 | 4 | 5.02 | N/A | 0.8 | 10 | LightScribe DVD
optical drive
| GO film | PET | In-plane | PVA/H2SO4 | 1 | 2.3 | 3.05 | 0.3 | 60 | Ref.70 | FS-IL | 2.5 | N/A | 2.35 | 2 | 150 | CO2 laser
| Hydrated GO film | Free-standing | In-plane | Hydrated GO | 1 | 0.51 | 3.1 | 0.43 | 1.7 | Ref.20 | CO2 laser
| PI | Free-standing | In-plane | H2SO4 | 1 | 4 | 1.5 | 0.3 | 50 | Ref.48 | BMIM-BF4 | 3.5 | 2 | 0.8 | 1 | 100 | fs 1030 nm laser | GO/HAuCl4 | Paper | In-plane | PVA/H2SO4 | 1 | 0.77 | 17.2 | N/A | N/A | Ref.71 | CO2 laser
| H3BO3/PI
| Free-standing | In-plane | PVA/H2SO4 | 1 | 16.5 | NA | 0.5 | 2 | Ref.72 | Laser-scribing DVD burner | CNT/GO | PET | In-plane | PVA/H3PO4 | 1 | N/A | 3.1 | 0.84 | 1 | Ref.73 | CO2 laser
| PI | Free-standing | In-plane | PVA/H2SO4 | 209 | N/A | 1.43×10-6 | 31.3 | N/A | Ref.74 | CO2 laser
| PI | Free-standing | Sandwich | PVA/H2SO4 | 1 | 9.11 | N/A | 3 | 2.5 | Ref.34 | CO2 laser
| GO film | Free-standing | In-plane | BMIM | 2.5 | 270 | N/A | 100 | 100 | Ref.75 | CO2 laser
| PI-LSG +Ni-CAT MOF | Free-standing | In-plane | PVA/LiCl | 1.4 | 15.2 | N/A | 4.1 | 7 | Ref.35 | CO2 laser
| PI-LSG +PANI | Free-standing | In-plane | PVA/H2SO4 | 0.8 | 361 | 47.5 | 1.1 | 1.511 | Ref.76 | PI-LSG +MnO2 | Free-standing | In-plane | PVA/LiCl | 1 | 934 | 93.4 | 3.2 | 0.298 | PI-LSG +FeOOH/LSG +MnO2 | Free-standing | In-plane asymmetric | PVA/LiCl | 1.8 | 21.9 | 5.4 | 2.4 | 2.891 | CO2 laser
| PI-LSG +Fe3O4/
LSG
| Free-standing | In-plane asymmetric | PVA/H2SO4 | 1 | 719.28 | 63.04 | 5.3 | 0.02648 | Ref.77 | 1064 nm laser | PI | Free-standing | In-plane | PVA/LiCl | 1 | 1 | N/A | 1 | 0.02 | Ref.78 | Sandwich | 34.77 | 10.21 | 1 | 0.005 | 355 nm ns laser | GO/Ni | PET | In-plane | PVA/LiCl | 1 | 3.9 | 0.693 | 5.7 | 3 | Ref.79 | 800 nm fs laser | GO film | Silicon oxide | In-plane | PVA/H2SO4 | 0.5 | 6.3 | 105 | 70 | 10 | Ref.80 | 800 nm fs laser | GO film | PDMS | In-plane 3D | FS-IL | 2.5 | 0.181 | 0.086 | 100 | 2200 | Ref.81 |
|
Table 1. The performances of laser scribed graphene based supercapacitors