
- Journal of Semiconductors
- Vol. 41, Issue 3, 032301 (2020)
Abstract
1. Introduction
III-nitride materials are direct bandgap semiconductors, the bandgap crosses from the infrared to the ultraviolet spectral range[
In order to expand the applications of GaN-based LEDs to display, visible light communication, optogenetics and other advanced applications, researchers are concentrating on developing high power and luminous efficiency III-nitride LEDs[
In this work, a hybrid white micro-LED array integration with inorganic quantum dots is designed and fabricated. Electrical and optical characterization of this hybrid white micro-LED array shows that the devices have a lower turn-on voltage and reverse leakage current. With the current density is increasing from 3.2 to 1114 A/cm2, and the peak of electroluminescence (EL) of the device has a small blue shift. The photoluminescence spectra (PL) of hybrid white LEDs was measured experimentally, and the calculated color temperature was 5596 K. This result indicates an effective way of achieving high-performance devices for illumination and display applications.
2. Device fabrication
The blue micro-LED array with micron-pillar structures is fabricated from planar LED wafers grown by metal organic chemical vapor deposition (MOCVD) on c-plane (0001) sapphire substrates. The epitaxial structure is shown in Fig. 1(a). Firstly, the micron-pillar array structures were formed by a standard lithography and inductively coupled plasma (ICP) etching process. By optimizing the etching conditions, we can reduce the side-wall damage caused by etching. The micron-pillars were etched through the P-type GaN layer to N-type GaN with depth of about 1.2 µm, as shown in Fig. 1(b). For this structure, the unit of the micro-LED array has a common N-contact electrode while the P-contact electrode is independent. A 100 nm SiO2 layer was developed by plasma enhanced chemical vapor deposition (PECVD) to use as a passivation layer for reducing leakage channels and protecting devices. Then the pattern of N-type and P-type electrodes are formed by photolithography and the N-type and P-type Cr/Au electrodes are fabricated by electron beam evaporation simultaneously, as shown in Fig. 1(c). The process of N-type and P-type electrodes in one-step evaporation simplifies the processes, compared to the fabrications of N- and P-contact metals, separately. Finally, the CIS ((CuInS2-ZnS)/ZnS) core–shell quantum dots with a luminescent peak wavelength of 580 nm dispersed in methylbenzene organic solution were spin-coated on the sample with micron-pillar arrays to fill the gaps between the micron-pillars. The sample is then placed on a hot table to evaporate the methylbenzene organic solvent to stabilize the quantum dots on the sample. By this way, we obtained the hybrid white micro-LEDs, as shown in Fig. 1(d).
Figure 1.(Color online) (a) Epitaxial structure of blue LED. (b) The micron-pillar array structures after lithography and ICP etching. (c) N-type and P-type Cr/Au electrodes are manufactured in only one step. (d) Micron-pillar structures with the CIS ((CuInS2-ZnS)/ZnS) core-shell quantum dots.
For device observations, a bird’s eye view microscope image of the blue micron-pillar LED array is shown in Fig. 2(a). Each cell of the array contains 16 × 16 pixels, and each pixel is a circle with a diameter of 20 µm with a period of 25 µm, and the diameter of the P-type electrodes is 10 µm, as shown in Fig. 2(b). Figs. 2(c) and 2(d) show the scanning electron microscope (SEM) image of the blue micro-LEDs array with micron-pillar structures. It can be seen that this micro-array has good periodicity and uniform size. As shown in Fig. 2(d), the etching depth of the micron-pillar is measured as ~ 1.2 μm as designed, and the sidewalls look straight and smooth, which indicates less etching damage after optimizations.
Figure 2.(Color online) (a) Bird’s eye view microscope image of the blue micron-pillar LED array. (b) The enlarged picture of micron-pillar structures. (c) SEM image of the blue micron-pillar LED array. (d) The cross-sectional view SEM image of blue micron-pillar LED array.
3. Results and discussion
Firstly, the current and voltage (I–V) characteristics of the hybrid white micro-LEDs array are analyzed, and the results are shown in Fig. 3(a). It can be obtained from the I–V curve that, the turn-on voltage of the hybrid LEDs is 3.5 V and the reverse leakage current is about 5 × 10–10 A (1.6 × 10–4 A/cm2) when the reverse voltage is 5 V. It should be pointed out that, the turn-on voltage of hybrid white micro-LED is close to that of the standard planar LEDs, and the reverse leakage current is on a smaller magnitude. It indicates that the device has a good electrical performance[
Figure 3.(Color online) (a)
Then the room temperature electroluminescence (EL) spectra of the hybrid white micro-LED arrays were acquired when the current density increased from 3.2 to 1114 A/cm2, the results are shown in Fig. 3(b). The emission peak of blue micro-LEDs moves slightly from 476 to 472 nm as the injection current density increases, it shows great emission wavelength stability. This is mainly attributed to the decreasing of quantum confined Stark effect (QCSE), consequently, the recombination efficiency of carriers gets a great increase[
Finally, the room temperature photoluminescence (PL) spectra of the hybrid white micro-LEDs were obtained using a Renishaw InVia Micro-PL system. From Fig. 4(a), we can see that the peak at 478 nm corresponds to the luminescence of InGaN/GaN MQWs, and the peak at 580 nm corresponds to the luminescence of CIS quantum dots. Both the luminescence sources combine to give a mixed nearly white color, the corresponding color coordinates of which are calculated to be (0.3303, 0.3501), and the color temperature is to be 5596 K. The data points in the CIE chromaticity diagram are shown in Fig. 4(b).
Figure 4.(Color online) (a) The PL spectra of CIS quantum dots and one pixel in the hybrid white micro-LEDs array. (b) The locations of hybrid white LEDs in the CIE 1931 chromaticity diagram.
4. Conclusions
In summary, the blue micro-LED array with micron-pillar structures have been fabricated using micromachining technology, and then integrated with 580 nm CIS quantum dots for white emissions and display application. The electrical properties of hybrid white micro-LEDs are manifested by I–V characteristics, which show good electrical performance. The EL spectra of the hybrid white micro-LEDs at the injection current density ranging from 3.2 to 1114 A/cm2 shows that the emission peak is stable, but still has a small blue shift due to the QCSE. From the PL spectra of the hybrid white micro-LED array, better white light emission with color coordinates of (0.3303, 0.3501) and color temperature of 5596 K have been achieved. This work provides an effective way for high-performance applications of illumination and display.
Acknowledgments
We would like to thank Prof. Haizheng Zhong and his graduate students at Beijing Institute of Technology, for providing high-quality CIS quantum dots. This work is supported by National Key R&D Program of China (2016YFB0400100), National Nature Science Foundation of China (61921005, 61674076, 61674081, 61605071, 61974062), Nature Science Foundation of Jiangsu Province (BY2013077, BK20141320, BE2015111), Six Talent Peaks Project of Jiangsu Province (XYDXX-081), Open Fund of the State Key Laboratory on Integrated Optoelectronics (IOSKL2017KF03), Innovation Project of Postgraduate Training in Jiangsu Province (KYCX18_0031), Fundamental Research Funds for the Central Universities (021014380096), Collaborative Innovation Center of Solid State Lighting and Energy-saving Electronics.
References
[1] S Nakamura, T Mukai, M Senoh et al. Candela-class high-brightness ingan/algan double-heterostructure blue-light-emitting diodes. Appl Phys Lett, 64, 1687(1994).
[2] H X Jiang, J Y Lin. Nitride micro-LEDs and beyond — a decade progress review. Opt Express, 21, A475(2013).
[3] J M Li, Z Liu, Z Q Liu et al. Advances and prospects in nitrides based light-emitting-diodes. J Semicond, 37, 061001(2016).
[4] J J D McKendry, D Massoubre, S Zhang et al. Visible-light communications using a CMOS-controlled micro-light-emitting-diode array. J Lightwave Technol, 30, 61(2012).
[5] H Qian, S Zhao, S Z Cai et al. Digitally controlled micro-LED array for linear visible light communication systems. IEEE Photonics J, 7, 7901508(2015).
[6] W R Liu, C W Yeh, C H Huang et al. (Ba, Sr)Y2Si2Al2O2N5: Eu2+: a novel near-ultraviolet converting green phosphor for white light-emitting diodes. J Mater Chem, 21, 3740(2011).
[7] H P T Nguyen, K Cui, S Zhang et al. Controlling electron overflow in phosphor-free InGaN/GaN nanowire white light-emitting diodes. Nano Lett, 12, 1317(2012).
[8] Z Zhuang, X Guo, B Liu et al. High color rendering index hybrid III-nitride/nanocrystals white light-emitting diodes. Adv Funct Mater, 26, 36(2016).
[9] T H Lin, S J Wang, Y C Tu et al. Enhanced light emission in vertical-structured GaN-based light-emitting diodes with trench etching and arrayed p-electrodes. Solid-State Electron, 107, 30(2015).
[10] M A Tsai, P Yu, C L Chao et al. Efficiency enhancement and beam shaping of GaN–InGaN vertical-injection light-emitting diodes via high-aspect-ratio nanorod arrays. IEEE Photonics Technol Lett, 21, 257(2009).
[11] G Tan, Y Huang, M C Li et al. High dynamic range liquid crystal displays with a mini-LED backlight. Opt Express, 26, 16572(2018).
[12] C Gossler, C Bierbrauer, R Moser et al. GaN-based micro-LED arrays on flexible substrates for optical cochlear implants. J Phys D, 47, 205401(2014).
[13] C L Qi, Y Huang, T Zhan et al. Fabrication and characteristics of excellent current spreading GaN-based LED by using transparent electrode-insulator-semiconductor structure. J Semicond, 38, 084005(2017).
[14] R Smith, B Liu, J Bai et al. Hybrid III-nitride/organic semiconductor nanostructure with high efficiency nonradiative energy transfer for white light emitters. Nano Lett, 13, 3042(2013).
[15] Q Sun, W Yan, M Feng et al. GaN-on-Si blue/white LEDs: epitaxy, chip, and package. J Semicond, 37, 044006(2016).
[16] W Chen, G Hu, J Lin et al. High-performance, single-pyramid micro light-emitting diode with leakage current confinement layer. Appl Phys Express, 8, 032102(2015).
[17] G Li, W Wang, W Yang et al. GaN-based light-emitting diodes on various substrates: a critical review. Rep Prog Phys, 79, 056501(2016).
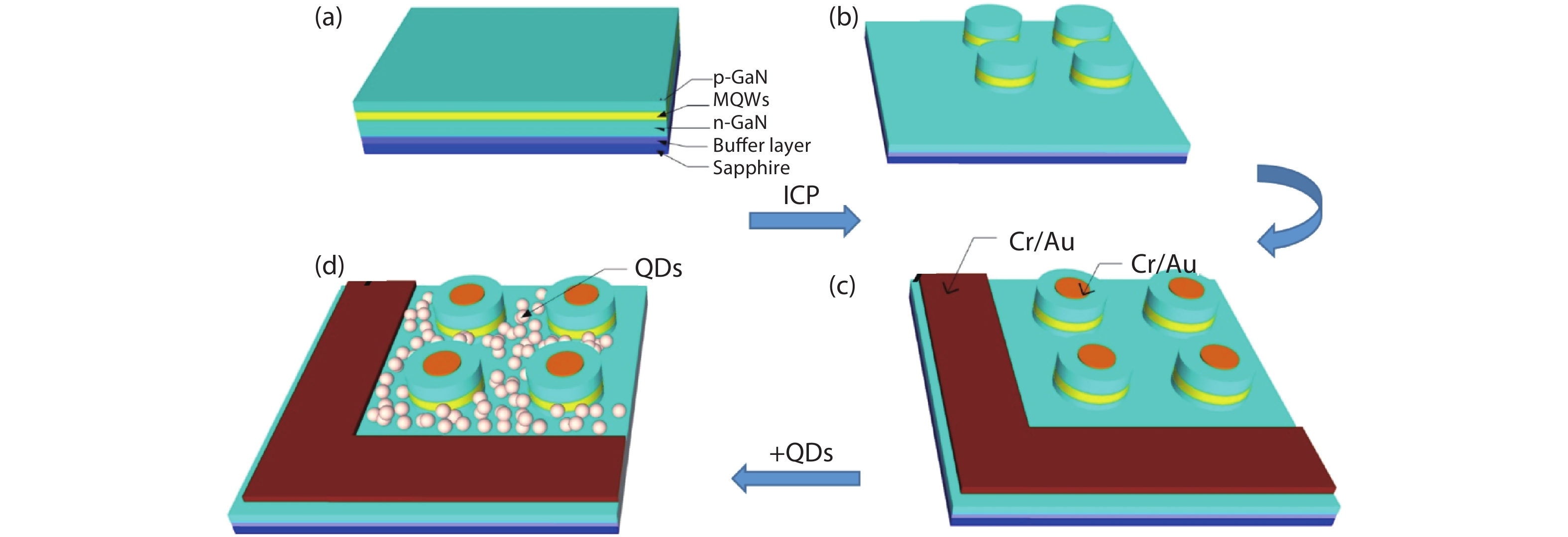
Set citation alerts for the article
Please enter your email address