Olena Kovalenko, Young-Sik Ra, Yin Cai, Vladyslav C. Usenko, Claude Fabre, Nicolas Treps, Radim Filip, "Frequency-multiplexed entanglement for continuous-variable quantum key distribution," Photonics Res. 9, 2351 (2021)

Search by keywords or author
- Photonics Research
- Vol. 9, Issue 12, 2351 (2021)
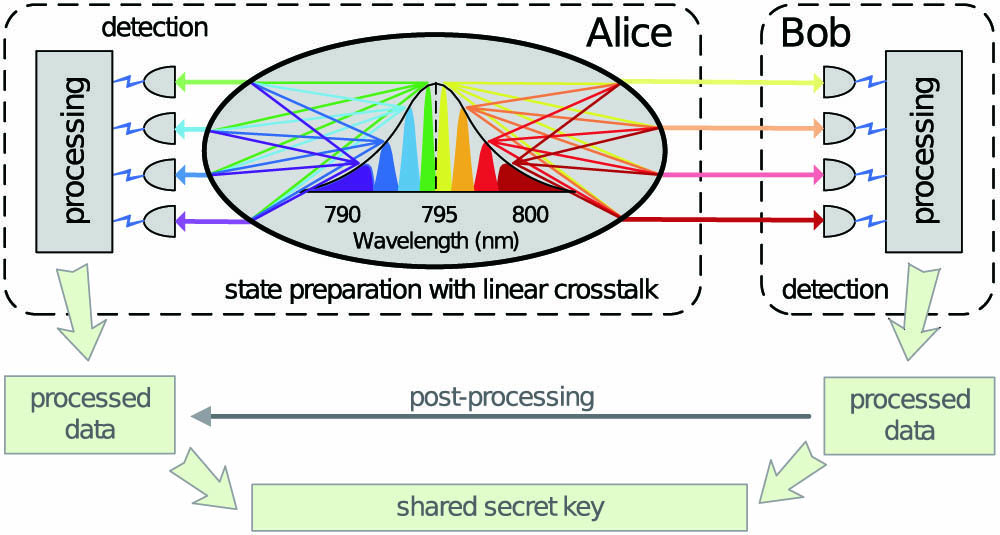
Fig. 1. Bright colors show a sketch of a CV QKD testbed for the study of the multimode entangled source at the side of the sender, Alice, with cross-talk coupling between the frequency modes in both of the two beams, leaving the source. The entangled source is based on eight pairs of modes, where only four of them are shown for clarity. We consider a scenario where half of the modes (below the central frequency) are locally measured by Alice and another half (above the central frequency) transmitted to a remote trusted party Bob (trusted devices are given in dashed blocks). Both multimode beams are detected by homodyne detectors and processed to optimally eliminate the cross talk and improve the secret key rate. The data processing corresponds to a local physical multimode symplectic transformation and was optimized to achieve higher key rate between the trusted parties. The trusted parties then can use authenticated classical channel to perform post-processing by correcting their errors and amplifying the data privacy in order to obtain quantum-secure key as the result (this part of the protocol was modeled numerically so is illustrated in pale colors).
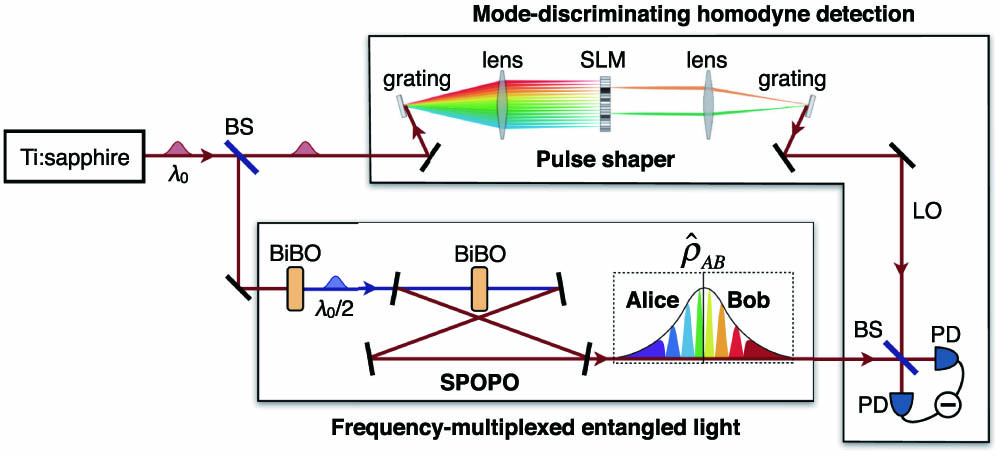
Fig. 2. Experimental setup for generation of frequency-multiplexed multimode entangled light and its measurement with mode-discriminating homodyne detection. The generated multimode light ρ ^ A B is in 16 frequency modes, where Alice (A) and Bob (B) access to the eight lower frequency modes and the other eight frequency modes, respectively. The pulse shaper is constructed in the folded configuration in actual implementation. BS, beam splitter; SLM, spatial light modulator; PD, photodiode. See main text for details.
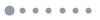
Fig. 3. Left panel: estimated key rate (in terms of bits per multimode channel use) of CV QKD based on the frequency-multiplexed entangled source in Fig. 1 for different number of pairs measured by Alice and Bob as obtained from the original data before decoupling (circles, blue lines) and after decoupling of modes involved in the multimode cross talk by optimized data processing performed by the trusted parties after the homodyne measurement (squares, yellow lines). The dotted, dotted–dashed, and dashed lines represent the pessimistic estimates that take into account standard error for the respective number of measurements N = 5 × 10 3 , N = 10 4 , N = 4 × 10 4 , as indicated over the lines in the plots (see Appendix A for details). Note that blue non-solid lines are almost extinct on the plot. Shaded areas represent the method prediction bands with 95% confidence level in the asymptotic limit of infinitely many measurement points. Realistic reconciliation efficiency β = 96 % taken for the processed data, perfect β = 1 taken for the original data. While multiplexing brings only small and fragile advantage when all the pairs are being used, it can be drastically improved by optimized local data manipulations, revealing power of frequency multiplexing in CV QKD. The improvement gets more pronounced as the number of data points N increases. Right panel: multimode mutual information (top) and Holevo bound (bottom) for different number of pairs measured by Alice and Bob as obtained from the original data (circles, blue line) and after optimized linear interactions performed by the trusted parties prior to the measurement (squares, yellow line). The plots illustrate the nature of improvement of frequency-multiplexed CV QKD by optimized data manipulations, which is based on increase of the mutual information, while the Holevo bound remains unchanged and, therefore, the yellow and blue points overlap.
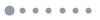
Fig. 4. Key rate of CV QKD versus channel transmittance T (in dB) as obtained from the original data on the full multimode entangled state (blue solid line), after optimized local data manipulations performed by the trusted parties for different number of used pairs of modes (non-solid lines for reduced number of pairs and thick solid violet line for the maximum number of eight pairs), linear extrapolation for larger number of modes (blue and brown dashed lines). Post-processing efficiency β = 96 % . Evidently, optimized data manipulation can drastically improve robustness to loss (and, respectively, the secure distance) of frequency-multiplexed CV QKD with entangled states.
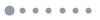
Fig. 5. Visualization of covariance matrices in x ^ quadrature (left) and p ^ quadrature (right) before (top) and after (bottom) the optimized linear data processing.
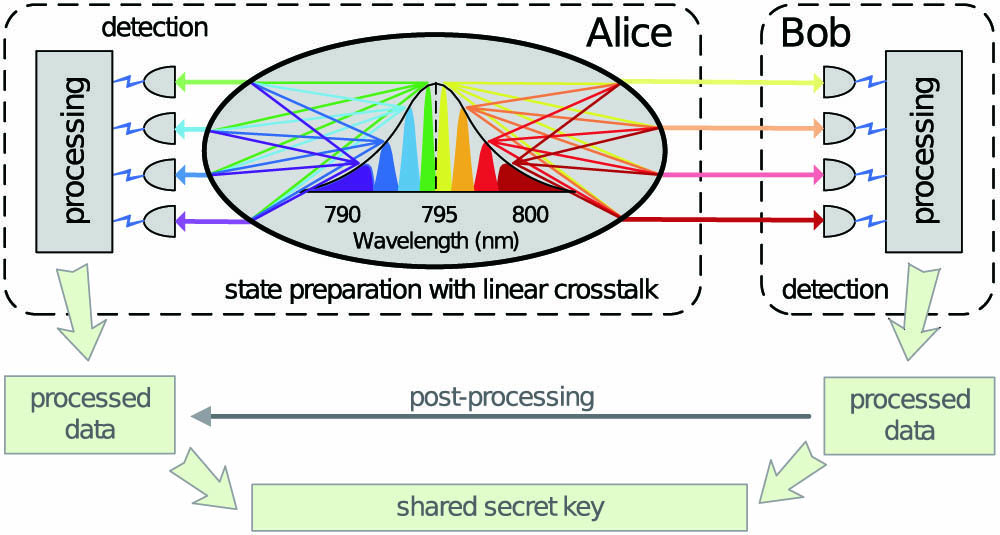
Set citation alerts for the article
Please enter your email address