
- High Power Laser Science and Engineering
- Vol. 7, Issue 3, 03000e48 (2019)
Abstract
1 Introduction
High-power laser facilities have been established for research on inertial confinement fusion (ICF), high-energy-density (HED) physics, laboratory-scale astrophysics, etc. [
2 Methods
2.1 Phase modulator design
Figure
Figure
Sign up for High Power Laser Science and Engineering TOC. Get the latest issue of High Power Laser Science and Engineering delivered right to you!Sign up now
2.2 Algorithm
Figure
(1) The
(2) The difference between the recorded diffraction pattern
(3) Application of modulus constraints with the recording pattern
Compared to single-wavelength CMI, we adopt a different Fourier constraint where the modulus of each wavelength is scaled by its real energy while its own phase is preserved. The denominator of Equation (
(4) Saturation processing is applied using Equation (
(5) Backpropagation of the light field of each wavelength to the modulator plane
(6) Backpropagation to the support plane
(7) Propagation of
3 Simulations and experiments
3.1 Simulation
The feasibility of our proposed method was initially examined using numerical simulations. Figure
Figure
Figure
By multiplying the complex amplitudes shown in Figure
Using the aforementioned reconstruction algorithm, the complex amplitudes of the three wavelengths can be iteratively determined, and their corresponding reconstructed accuracy can be calculated using Equation (
The change in the corresponding reconstruction error for each wavelength throughout the iteration process is represented in Figure
To further demonstrate the feasibility and reliability of our method, we added another numerical simulation with different lens aberrations for each wavelength. Figure
Figures
Using our proposed method, the reconstructed results are shown in Figure
3.2 Experiments
Our experimental setup shown in Figure
The transmission functions of the modulator for each wavelength were characterized by ePIE (extended ptychographic iterative engine)[
Figure
To examine the measurement accuracy of the aforementioned experiment, several experiments were performed to separately measure the complex amplitude of each wavelength using the conventional CMI method. By the introduction of an appropriate filter behind the tripler to allow only one wavelength to form diffraction patterns on the detector at a time, the diffraction patterns associated with the three wavelengths can be separately recorded. Subsequently, the conventional single-wavelength CMI algorithm can be utilized to reconstruct their complex amplitudes. The reconstructions are shown in Figure
Figures
Additional calculations were performed to quantitatively analyze the reconstruction errors further. The reconstruction errors were computed using Equation (
The spatial resolution and measurement accuracy of the proposed three-wavelength CMI approach were also studied. A phase resolution target with a structure similar to the common USAF 1951 pattern was used. However, in this case, each tiny bar was evaporated silicon on a quartz plate and the transmitted phase retardation was previously calibrated using an interferometer. Thus, this kind of phase resolution target can be used to simultaneously check the capability of three-wavelength CMI in terms of spatial resolution and phase measurement accuracy. In the experiments, one diffraction pattern
4 Conclusion
To address the problem of simultaneously measuring the fundamental, second and third harmonics behind FOAs for high-power laser facilities, a three-wavelength CMI-based online diagnosis technique was proposed. A specially designed modulator was adopted to scatter the hybrid laser beam and produce diffraction patterns, and a conventional CMI algorithm was modified to simultaneously reconstruct the complex amplitude of each wavelength. The feasibility of this proposed method was demonstrated both numerically and experimentally. The spatial resolution and measurement accuracy can approach
References
[1] R. Betti, O. A. Hurricane. Nat. Phys., 12, 435(2016).
[2] E. I. Moses, E. Storm. Epj Web Conf., 59(2013).
[3] D. W. Yuan, H. G. Wei, G. Y. Liang, F. L. Wang, Y. T. Li, Z. Zhang, B. J. Zhu, J. R. Zhao, W. M. Jiang, B. Han, X. X. Yuan, J. Y. Zhong, X. H. Yuan, C. B. Fu, X. P. Zhang, C. Wang, G. Jia, J. Xiong, Z. H. Fang, S. Jiang, K. Du, Y. K. Ding, N. Hua, Z. F. Qiao, S. L. Zhou, B. Q. Zhu, J. Q. Zhu, G. Zhao, J. Zhang. High Power Laser Sci. Eng., 6, e45(2018).
[4] A. Casner, G. Rigon, B. Albertazzi, T. Michel, T. Pikuz, A. Faenov, P. Mabey, N. Ozaki, Y. Sakawa, T. Sano, J. Ballet, P. Tzeferacos, D. Lamb, E. Falize, G. Gregori, M. Koenig. High Power Laser Sci. Eng., 6, e44(2018).
[5] E. I. Moses, J. D. Lindl, M. L. Spaeth, R. W. Patterson, R. H. Sawicki, L. J. Atherton, P. A. Baisden, L. J. Lagin, D. W. Larson, B. J. MacGowan, G. H. Miller, D. C. Rardin, V. S. Roberts, B. M. Van Wonterghem, P. J. Wegner. Fusion Sci. Technol., 69, 1(2016).
[6] C. A. Haynam, P. J. Wegner, J. M. Auerbach, M. W. Bowers, S. N. Dixit, G. V. Erbert, G. M. Heestand, M. A. Henesian, M. R. Hermann, K. S. Jancaitis, K. R. Manes, C. D. Marshall, N. C. Mehta, J. Menapace, E. Moses, J. R. Murray, M. C. Nostrand, C. D. Orth, R. Patterson, R. A. Sacks, M. J. Shaw, M. Spaeth, S. B. Sutton, W. H. Williams, C. C. Widmayer, R. K. White, S. T. Yang, B. M. Van Wonterghem. Appl. Opt., 46, 3276(2007).
[7] G. H. Miller, E. I. Moses, C. R. Wuest. Nucl. Fusion, 44, S228(2004).
[8] J. Q. Zhu, X. L. Xie, M. Z. Sun, J. Kang, Q. W. Yang, A. L. Guo, H. D. Zhu, P. Zhu, Q. Gao, X. Liang, Z. R. Cui, S. H. Yang, C. Zhang, Z. Q. Lin. High Power Laser Sci. Eng., 6, e29(2018).
[9] Y. Q. Gao, W. X. Ma, B. Q. Zhu, Z. D. Cao, J. Zhu, X. D. Yang, Y. P. Dai, Z. Q. Lin. IEEE Photonics Conference, 73(2013).
[10] J. L. Miquel, C. Lion, P. Vivini. J. Phys. Conf. Ser., 688(2016).
[11] P. Vivini, M. Nicolaizeau. Proc. SPIE, 9345(2015).
[12] Z. Y. Jiao, P. Shao, D. F. Zhao, R. Wu, L. L. Ji, L. Wang, L. Xia, D. Liu, Y. Zhou, L. J. Ju, Z. J. Cai, Q. Ye, Z. F. Qiao, N. Hua, Q. Li, W. Pan, L. Ren, M. Y. Sun, J. Q. Zhu, Z. Q. Lin. High Power Laser Sci. Eng., 6, e14(2018).
[13] P. Wegner, J. Auerbach, T. Biesiada, S. Dixit, J. Lawson, J. Menapace, T. Parham, D. Swift, P. Whitman, W. Williams. P. Soc. Photo-Opt. Ins., 5341, 180(2004).
[14] R. A. Zacharias, N. R. Beer, E. S. Bliss, S. C. Burkhart, S. J. Cohen, S. B. Sutton, R. L. Van Atta, S. E. Winters, J. T. Salmon, C. J. Stolz, D. C. Pigg, T. J. Arnold. Proc. SPIE, 5341, 168(2004).
[15] J. Bromage, S. W. Bahk, D. Irwin, J. Kwiatkowski, A. Pruyne, M. Millecchia, M. Moore, J. D. Zuegel. Opt. Express, 16(2008).
[16] J. R. Fienup. Opt. Lett., 3, 27(1978).
[17] J. R. Fienup. Appl. Opt., 21, 2758(1982).
[18] J. W. Miao, P. Charalambous, J. Kirz, D. Sayre. Nature, 400, 342(1999).
[19] S. W. Bahk, J. Bromage, I. A. Begishev, C. Mileham, C. Stoeckl, M. Storm, J. D. Zuegel. Appl. Opt., 47, 4589(2008).
[20] S. Matsuoka, K. Yamakawa. J. Opt. Soc. Am. B, 17, 663(2000).
[21] F. C. Zhang, J. M. Rodenburg. Phys. Rev. B, 82(2010).
[22] F. C. Zhang, B. Chen, G. R. Morrison, J. Vila-Comamala, M. Guizar-Sicairos, I. K. Robinson. Nat. Commun., 7(2016).
[23] X. Pan, S. P. Veetil, C. Liu, H. Tao, Y. Jiang, Q. Lin, X. Li, J. Zhu. Laser Phys. Lett., 13(2016).
[24] H. Tao, S. P. Veetil, X. C. Pan, C. Liu, J. Q. Zhu. Appl. Opt., 54, 6632(2015).
[25] X. Dong, X. C. Pan, C. Liu, J. Q. Zhu. Opt. Lett., 43, 1762(2018).
[26] A. M. Maiden, J. M. Rodenburg. Ultramicroscopy, 109, 1256(2009).
[27] F. C. Zhang, I. Peterson, J. Vila-Comamala, A. D. F. Berenguer, R. Bean, B. Chen, A. Menzel, I. K. Robinson, J. M. Rodenburg. Opt. Express, 21(2013).
[28] A. M. Maiden, M. J. Humphry, M. C. Sarahan, B. Kraus, J. M. Rodenburg. Ultramicroscopy, 120, 64(2012).
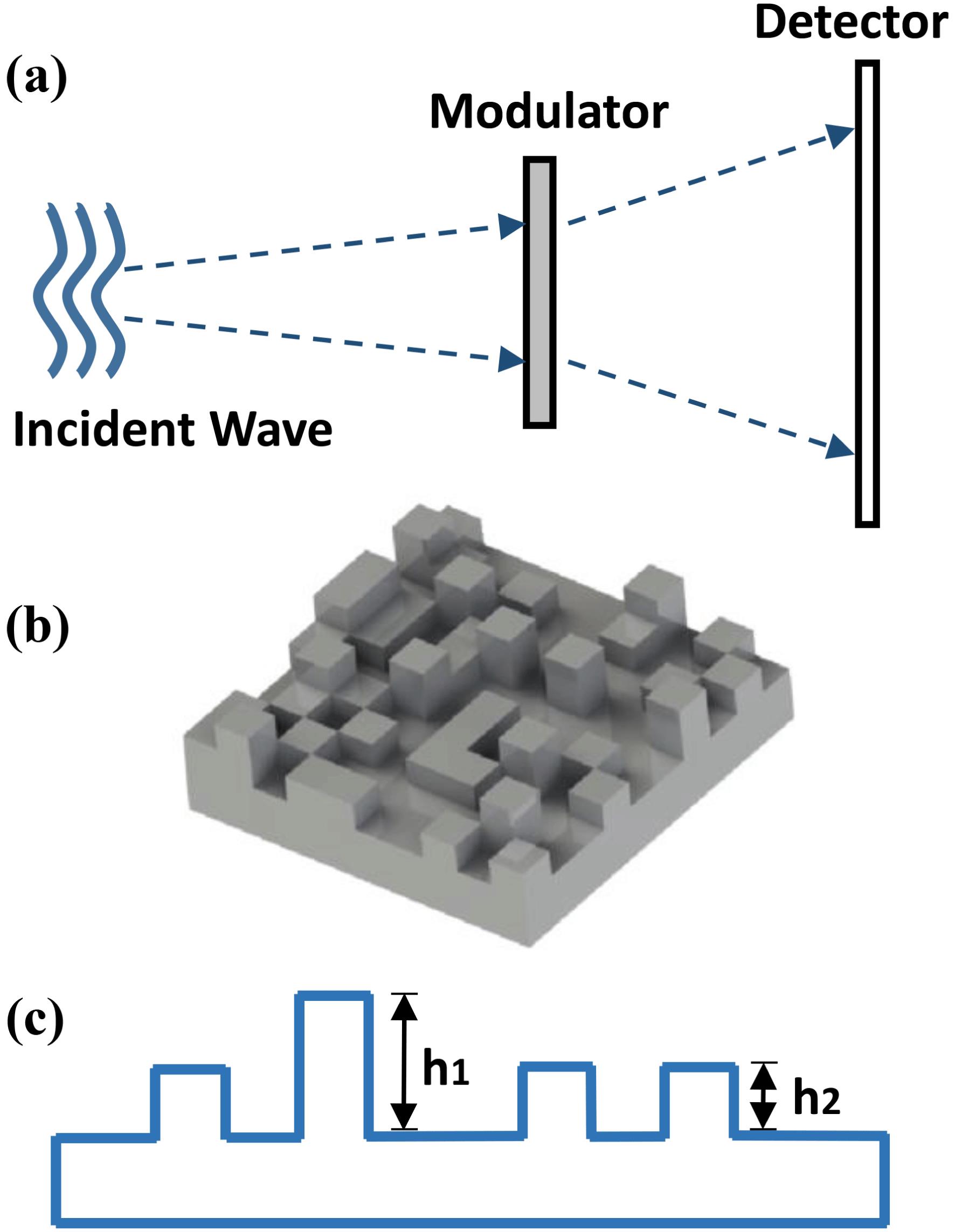
Set citation alerts for the article
Please enter your email address